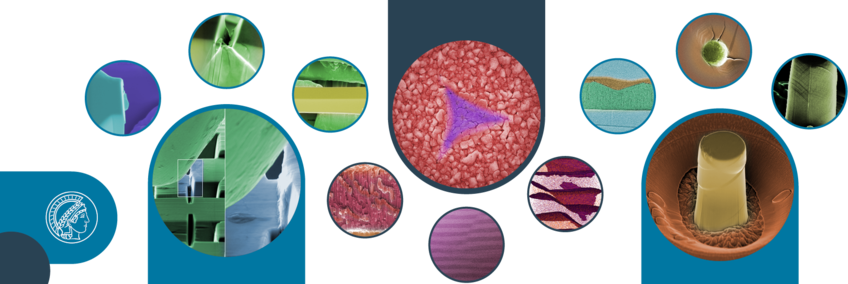
Nano- and microscale plasticity
Material strength and underlying plasticity mechanisms can change when deformed at micro- and nanometre length-scales. While being of fundamental scientific interest, such observations have significant implications for the design of advanced material systems, as for thin-films.
Nano- and microscale plasticity is crucial for the design and engineering of advanced materials with tailored mechanical properties. In nanomaterials, such as nanoparticles and nanocomposites, unique mechanical phenomena emerge due to the dominance of surface effects, influencing their strength, hardness, and ductility. Similarly, at the microscale, materials often exhibit size-dependent plasticity, with grain boundaries and interfaces significantly affecting mechanical response. The study of plasticity at these scales thus offers a gateway to harnessing unique material behaviours, driving innovation and advancement in diverse technological fields.
Dislocation interaction with grain boundaries in Cu: Grain boundaries (GBs) are not necessarily hard barriers to dislocation motion. Former PhD student Reza Hosseinabadi has looked at the transmission of dislocations through twin boundaries in Cu bicrystals, where the barriers to transmission are very small. In his work, size effects on transmission were studied through variation of the pillar diameter where curvature of the dislocation line before cross-slip over the boundary was important (Acta Materialia, 230, 2022, 117841; Materials & Design, 232, 2023, 112164). PhD student Hendrik Holz follows these ideas on, by “tuning” the thermal barriers for dislocation/GB interaction by testing under cryogenic temperatures and high strain rates at different grain boundaries including low angle grain boundaries, working together with Dr. Raj Ramachandramoorthy. To suppress thermal activation, a set-up which can test at ultra-high strain rates will be combined with a custom build cryogenic system. Regarding the influence of GB chemistry on mechanical behaviour, PhD student Kamran Bhat works on the GB-CORRELATE ERC project of Prof. Dehm. For this purpose, we use Cu-Ag as a model system and compare the mechanical response/deformation behaviour of pure Cu bicrystals to that of Ag segregated Cu bicrystals. The Cu-Ag system is common in electronic components to prevent electromigration, and as Ag is almost immiscible in the Cu matrix it preferentially segregates to GBs and allows us to isolate the GB chemistry and its effect on the mechanical response. We utilise in situ micropillar compression coupled with nanoindentation, complimented by atom probe tomography and TEM analyses, and see significant effects of Ag-segregation to GBs on the strength (Acta Materialia, 255, 2023, 119081).
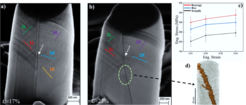
The concepts determined by testing bicrystals of Cu are extended to thin-films of Ag-doped Cu, containing a complex nanocrystalline nanotwinned structure. The segregation of Ag to Cu twin/GB nodes through mild heat treatment leads to an increase of the strength, where through collaboration with Dr. Tobias Brink we seek to understand and better describe mechanistically dislocation/GB interactions by atomistic simulations.
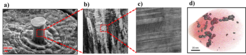
The unusual mechanical behaviour of B2 FeAl compounds: Fe-Al-based alloys with maximum Al contents of 50 at.% are regarded as potential structural material candidates for applications up to 800 °C owing to their excellent corrosion resistance, wear-resistance, relative low density, and comparably lower cost than high-alloyed steels or Ni-base superalloys. Nevertheless, B2-ordered FeAl alloys possess constitutional and thermal vacancies, which subsequently affect the alloy strength. Consequently, a direct evaluation and comparison of the mechanical properties of B2 FeAl alloys is in principle not possible if the alloys have different thermal histories or processing routes. Therefore, the aim of PhD student Jung-Soo Lee’s project, in a DFG-funded collaboration with Dr. Frank Stein and KIT, is to measure and compare the mechanical properties of different compositions of FeAl alloys using in situ nano- and micromechanical techniques by producing them in a single diffusion couple specimen. Based on this research, we aim to reveal the fundamental interrelation of the parameters (Al content, vacancy concentration, temperature etc.) on the mechanical properties, and couple to the results on larger specimens at KIT.
High-temperature deformation of iron oxide single crystals: In hydrogen-based direct reduction processes, iron oxide phases such as hematite and magnetite serve as the primary feedstock, with Wüstite acting as an intermediate phase, resulting in reduced alpha iron. However, contemporary solid-state reactors face notable challenges including excessive particle adhesion at temperatures surpassing 750°C, and the formation of a reaction layer on the outer surface, often leading to an unreacted Fe2O3 core, undermining process efficiency. Efforts to design future reactors must focus on promoting controlled fracture and delamination at the weakened metal/oxide interface induced by hydrogen. Nonetheless, there remains a considerable gap in understanding the micromechanical properties of iron oxide phases at elevated temperatures (600-800°C) and small length scales. The FeOx-HOTMECH project, funded by the KSB-Stiftung and undertaken by PhD student Shreehard Sahu, aims to elucidate the plasticity mechanisms of iron oxide phases in small volumes using nanoindentation and micropillar compression techniques, extending up to 800°C. This research will also investigate the brittle-to-ductile transition through microcantilever fracture analysis and further link with the plasticity mechanism. The findings from this project will provide crucial material parameters essential for the design of next-generation fluidised bed ironmaking processes with reduced environmental impact. Moreover, these insights will also facilitate the optimisation of ore grinding processes before fluidisation or pelletisation, as precise measurements of the fracture toughness of iron ores hold significant scientific and industrial relevance.