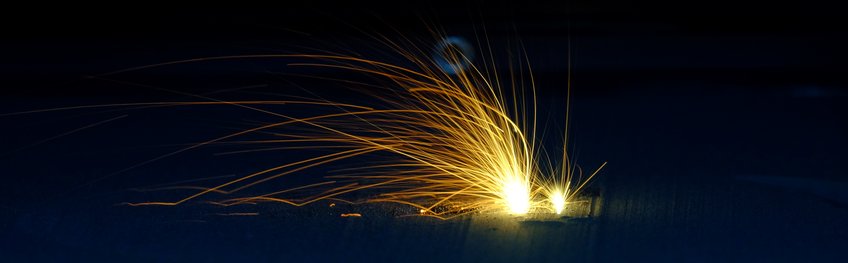
Interdisciplinary Research Key Topics
We analyse materials down to their atomic and electronic scale to unravel the correlation between their microstructure and properties. This comprehension empowers us to engineer sustainable and smart materials capable of enduring extreme operational environments. Employing state-of-the-art microscopy methods and computer simulations, we push the boundaries of material science. Our four departments collaborate with each other as well as with global partners to tackle the following pivotal research areas.