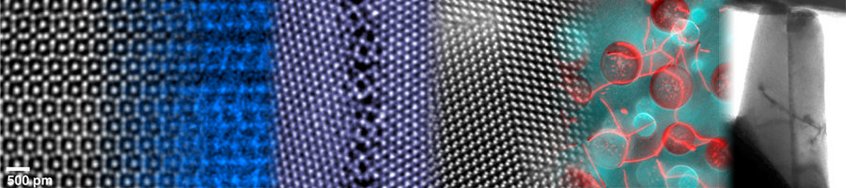
The atomic structure and transitions of grain boundaries in copper
Grain boundaries (GBs) are one of the most important features in metals regarding strength, microstructural stability or corrosion resistance. GB engineering has an enormous potential to improve material’s properties by controlling their microstructure. Structural transformations of GBs have been already suggested to affect the diffusivity or grain growth in a material. Nevertheless, few experimental studies explore the atomic structure of GBs, their transitions and draw correlations to materials properties.
Recently, it has been shown that textured copper (Cu) films are ideally suited to study pure tilt GBs, which can be lifted out by focused ion beam (FIB) preparation and then analyzed by aberration-corrected (scanning) transmission electron microscopy ((S)TEM). In a previous study, a GB phase transformation was revealed in a Σ19b [11-1] tilt GB where two different GB phases with different atomic structures were observed at room temperature by aberration-corrected STEM [1].
In a related Σ37c [11-1] tilt GB in Cu, we observed similar GB phases with an unexpected arrangement within the GB plane. Here, the GB is patterned by its two GB phases, the domino phase highlighted in red and the pearl phase indicated in blue in Fig.1. Interestingly, both phases form alternating patches along the slightly curved GB segment (Fig. 1a) with segment length between ~10 nm and ~50 nm. A closer inspection of the GB phases reveals that they are separated by a 1D line defect the GB phase junction indicated in yellow in Fig. 1b. The atomic resolution observations enabled us to determine the in-plane character of the phase junction, which is in agreement with atomistic simulations. Molecular dynamics (MD) simulations and thermodynamic integration find that the experimentally observed GB phases undergo a first order phase transformation (Fig. 1c) and reveal that the dislocation character of the GB phase junction plays an important role in the experimentally observed patterning of the GB at room temperature. This work provides unprecedented views into the intrinsic mechanisms of GB phase transitions in simple elemental metals and opens entirely novel possibilities to kinetically engineer interfacial properties. In a next step, the impact of solute segregation on the GB phase transition as well as their dynamic evolution under in situ conditions will be explored in the STEM. These insights might pave the way to develop novel materials with improved properties such as elevated strength and fracture toughness.
![Fig.1 a Overview of a 150nm-long segment of a Σ37c [11-1] {1 10 11} GB in Cu showing the alternation of domino (red) and pearl (blue) GB phases. b Both phases -separated by a GB phase junction- are revealed on the atomic level. c Free energy calculations of both GB phases indicate a phase transition at about 460 K from the high temperature domino to the low temperature pearl phase. The insets show the GB phase structures predicted by molecular statics simulations at 0K. The predicted GB structures perfectly match to the experimentally observed ones shown in Fig. 1b.](/4759738/original-1647256136.jpg?t=eyJ3aWR0aCI6MjQ2LCJvYmpfaWQiOjQ3NTk3Mzh9--eea04e3360a95214b83d8c3b7ba4c8459617e8cd)