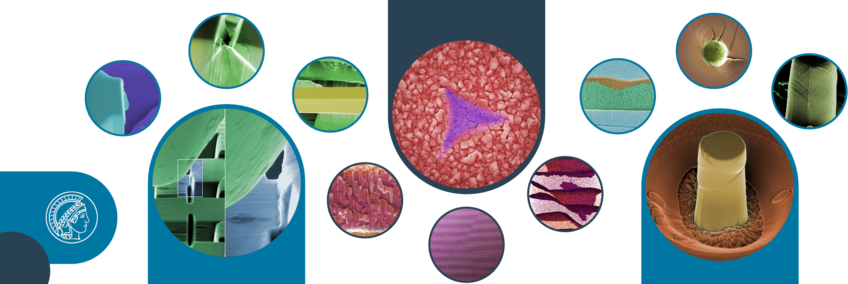
Interfaces in energy materials
Interfaces in energy materials have a profound impact on performance and reliability. In the realm of energy storage and conversion devices, such as batteries, fuel cells, and solar cells, interfaces play a critical role in governing key processes like charge transfer, ion diffusion, and electrochemical reactions.
The mechanical behaviour at these interfaces is closely linked to the structural stability and durability of the materials, which are crucial for long-term operation under varying conditions. Understanding the mechanics of interfaces enables the identification and mitigation of failure mechanisms, such as cracking, delamination, or stress-induced degradation, ultimately leading to the development of materials with enhanced mechanical integrity. Moreover, comprehending the interplay between mechanics and chemistry at interfaces empowers researchers to optimise material design, tailor interfacial properties, and unlock the full potential of energy materials, driving advancements towards sustainable and efficient energy solutions.
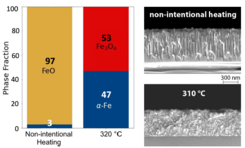
Defects in electrode materials: The electrochemical performance of energy materials are strongly influenced by defects such as interfaces, dislocations, and grain boundaries. These defects can alter the diffusion pathways for ions and electrons, affecting the overall charge storage and transfer kinetics, which directly impact efficiency, cycling stability, and lifespan. For water electrolysis, iron oxides have garnered significant attention as model electrode materials for the oxygen evolution reaction (OER). In recent years, the relationship between the microstructure of electrode materials and their electrochemical performance during OER have been studied. In particular, defect engineering offers the ability to fine-tune the OER activity of electrodes where surface defects, such as oxygen vacancies or edge dislocations, can serve as active sites for the OER reaction. Nina Nicolin, supported by the IMPRS SusMet program and in collaboration with Prof. Kristina Tschulik, looks at defects in reactive magnetron sputtered iron oxide thin-films as model spinel materials to investigate the influence of the microstructure on the catalytic performance during OER (S Evertz et al 2024 J. Phys. D: Appl. Phys. 57 065302). The connection between structural and compositional details and electrochemical performance will be studied using local analysis techniques such as scanning electrochemical cell microscopy (SECCM) to obtain novel insights into the role of defects on electrochemical performance.
Failure in solid-state lithium ion battery systems: Solid-state batteries promise to enable a variety of safer battery technologies. However, the rigidity of an all-solid-state battery poses significant challenges to maintaining mechanical and chemical integrity during manufacture and operation. Concerning the mechanical aspects, the process of anode metal stripping and cathode lithiation leads to volumetric changes in the electrode, resulting in the presence of gaps, stress accumulation, and solid electrolyte (SE) fracture. With regards to the chemical aspects, the substantial deterioration of the solid electrolytes occurs due to direct interaction with the active materials, e.g., the formation of ionic insulating layers at the cathode/SE and the formation of metallic Li within SE due to reduction of lithium ions at the GB. The aforementioned chemo-mechanical instability results in interfacial failure between the cathode/SE and anode/SE, which leads to a substantial reduction in the performance of all-solid-state batteries. In response to these challenges, most “solid-state” cells (in both industry and academia) make use of liquid electrolytes to maintain electrode/electrolyte contact. However, the use of liquid electrolytes undermines the safety advantages associated with non-flammable solid electrolytes. To fully harness the potential of solid-state batteries, Dr. Yuwei Zhang and students from Prof. Jennifer Rupp’s group at TU Munich are trying to address the chemo-mechanical compatibility issues at the interface of active material/SE. Till Freieck supports this work with environmental SEM analysis.
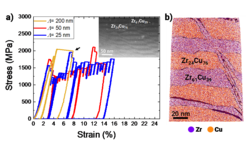
Nanostructured thin-films for electronics: Thin-films are crucial for electronics applications due to their ability to provide compact, lightweight, and flexible components, enabling the development of portable devices and wearable electronics. Additionally, thin-film technologies allow for precise control over material properties, making them essential for fabricating high-performance electronic devices with enhanced functionality and efficiency. We recently reported on Fe-doped Cu thin-films produced by magnetron sputtering, where the Fe localisation to grain boundaries could be controlled by thermomechanical processing with effects on the electrical conductivity (Scripta Materialia, 230, 2023, 115393). Recent work from the group has looked at nanostructuring and resulting effect on mechanical properties in amorphous Ni-P thin-films which have applications as metallisation layers in power electronics devices. Andrea Brognara and visiting PhD student Cristiano Poltronieri have also focussed on amorphous thin-films, but magnetron sputtered thin-film metallic glasses (TFMGs). For this project, in collaboration with Matteo Ghidelli from Sorbonne Paris Nord and supported by the DAAD and CRNS Salto program, we sought to understand how the local chemistry and structure of TFMGs affects mechanical and electrical performance (Materials & Design, 219, 2022, 110752). The activation of mechanical size effects TFMGs have been found to be an effective way to improve plastic deformation and strain-to-failure of these amorphous alloys, while the introduction of interfaces within the glass structure has been shown to further improve the mechanical properties by hindering the propagation of shear bands (SBs, see SmallStructures, 2024, 2400011). Indeed, for our systems micropillar compression shows that the bilayer period mediates the deformation mode, from the formation of single SBs and consequent catastrophic brittle failure to SB multiplication. Furthermore, fully amorphous multilayered ZrCu/ZrCuAl TFMGs were deposited by magnetron co-sputtering with different bi-layer periods and tested mechanically. Despite the similar elastic properties of the two monolithic materials, the crucial effect of the bilayer period was also observed on failure (Acta Materialia, 258, 2023, 119226). Overall, nanostructuring shows to be an effective strategy for tuning the behaviour of both crystalline and amorphous metallic thin-films, and is the focus of further work on high entropy alloy thin-films with visiting PhD student Davide Vacirca.