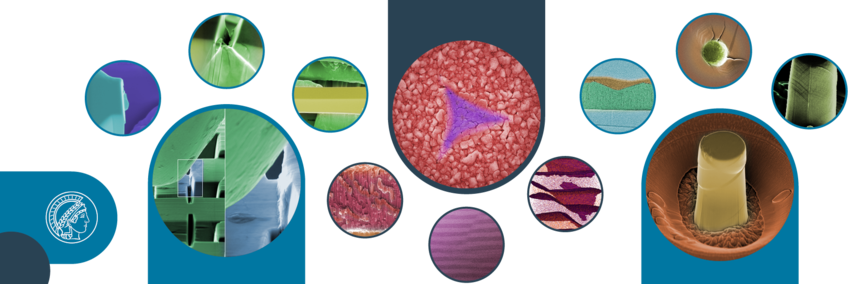
Fracture at interfaces
Our focus lies on investigating the fracture of materials down to individual microstructural length-scales, with special attention on grain/phase boundaries or material interfaces, while considering the role of crystallography, chemistry and non-ambient conditions such as high temperatures and cryogenic conditions on fracture.
Whether macroscale components for structural applications such as bridges, ships, aircraft, or microscale systems such as thin-films or interconnects in microelectronic devices, fracture is largely governed by interactions between growing crack tips and microstructural interfaces. A prime example is intergranular stress corrosion cracking, where cracks preferentially follow chemomechanically affected grain boundaries. The primary goal of this research theme is to investigate the fracture properties of materials down to the individual microstructural length scale. In our group, we probe the site-specific fracture properties through in situ small-scale testing methods inside a scanning electron microscope to glean novel insights into material failure.
Chemical effects on interfacial fracture: The segregation of chemical species to low-energy sites, such as grain boundaries (GBs) can have a pronounced effect on fracture along these interfaces. In collaboration with the FZ Jülich, together with molecular dynamics (MD) support from Prof. Erik Bitzek, we have studied in detail the embrittlement of recrystallised Tungsten using site-specific microcantilever tests where the sharp notch used to initiate fracture was localised directly at the intersection of GBs with the surface. This work was funded by the ERC projects of both Prof. Bitzek and Prof. Dehm. Using our novel micromechanical tests coupled with atom probe tomography, we were able to demonstrate that during recrystallisation and grain growth, Phosphorous segregates to GBs and leads to a clear embrittlement of the W (Acta Materialia, 259, (2023) 119256). Leveraging similar concepts, and in collaboration with Dr. Xufei Fang, we have also investigated the high-temperature oxidation of a Fe-Cr alloy where significant oxygen accumulates at GBs using novel in situ approaches to study local fracture at these interfaces (Corrosion Science, 225, (2023) 111613).
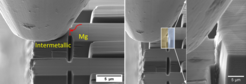
(see Acta Materialia 259 (2023) 119256)
Fracture of intermetallic materials, including at high-temperatures: In research led by Dr. Anwesha Kanjilal, and supported by the DFG through SFB1394, we investigate the role of crystallography and composition on the fracture of intermetallic materials. Many metallic alloys have microscale intermetallic precipitates dispersed in their matrix, which may improve the strength of the alloy but their brittle nature also makes them susceptible to cracking and fracture. Through micro- and nano-mechanical testing we investigate the fracture toughness of Laves phases in the Mg-Al-Ca system, while additionally highlighting the role of temperature and cleavage planes, which are then used to understand the interface strength between the Laves phase and Mg. Cubic C15 CaAl2 and hexagonal C14 CaMg2 Laves phases are studied as single-phase specimens, which are commonly found in Mg-alloys. Microscale fracture testing based on microcantilever bending and micropillar splitting are used to determine the toughness, while cleavage planes are initially identified through spherical indentation assisted by MD simulations in collaboration with the group of Prof. Bitzek (arXiv:2403.09461 [cond-mat.mtrl-sci]). We employ high-temperature nanoindentation to monitor changes in slip activity and cracking and screen the transition temperature from brittle to ductile behaviour for the Laves phases, where results are put into context of high-temperature plasticity measurements from collaborators at the RWTH Aachen (arXiv:2403.12507v1 [cond-mat.mtrl-sci]). The effect of temperature on fracture toughness is evaluated using microscale fracture testing techniques, while the composition is additionally varied using combinatorial thin films of the Laves phases, allowing us to obtain fundamental insights into the role of crystallography and chemistry on fracture properties of these materials. Additionally, the strength of intermetallic-metal interface is being investigated using a novel approach which involves localised microshear testing of the CaMg2-Mg interface prepared using combinatorial thin films of the Laves phase intermetallic deposited on Mg. Finite element modelling is also employed to optimise the microshear specimen dimensions and obtain geometry insensitive interface strength. This simple technique allows direct quantification of the strength of intermetallic-metal interfaces.
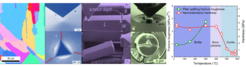
(see Journal of Materials Science, Metals & corrosion)
Low-temperature interfacial fracture: Fracture under cryogenic or low-temperature conditions has been observed in cases such as the failure of Liberty ships, the Challenger space shuttle disaster, sinking of the Titanic due to the hard glacier ice, or even of glaciers. It is essential to understand low-temperature deformation and failure to avoid such catastrophic events, as material brittleness generally increases at lower temperatures. In addition to collaborations with Prof. Vera Popovich of TU Delft investigating low-temperature fracture of high strength steel containing high intermetallic fractions, we also focus on developing experimental methodologies to investigate the nanomechanics and fracture of frozen liquids, such as water ice (see PLoS ONE 18(2): e0281703). This work on the in situ mechanical testing on ice is led by PhD student Taulant Sinani, co-supervised by Prof. Baptiste Gault and supported by the Leibniz prize of Prof. Gault. The fracture of water ice is highly affected by chemical impurities, like salts, which strongly segregates to GBs. However, intergranular failure and chemical heterogeneities are not considered in current glacial models. To correct this, we are developing a cutting-edge low-temperature nanoindentation setup featuring Peltier coolers to examine real-time ice deformation within our environmental SEM. This innovative system provides precise temperature control, facilitating direct microscale investigations into ice mechanics in situ. Given that GBs serve as interfaces where soluble impurities like Na, K, Cl, Mg, and S and others tend to segregate, understanding how these impurities impact fracture at GBs is crucial. This insight can greatly enhance models of glacier flow in natural environments. In our work, ice samples with different cooling rates (affects the grain size and gas bubble frequency) and impurity concentration are being prepared for correlating the fracture with the chemistry at GBs.
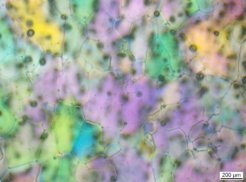