Developing high-strength and damage-tolerant crystalline thin-film materials
Wear-related energy loss and component damage, including friction and remanufacturing of components that failed by surface contacts, has an incredible cost. While high-strength materials generally have low wear rates, homogeneous deformation behaviour and the accommodation of plastic strain without cracking or localised brittle fracture are also crucial for wear-resistant metals.
High-strength and tough coatings have a large role to play within this space, and in this interdepartmental project we have developed both techniques such as high-throughput tensile testing [1], and advanced materials systems such as MAX phase Cr2AlC coatings [2] and novel interstitial solid solution strengthened thin films [3], to address this challenge.
Amorphous-crystalline nanocomposite thin-films have also been found to promote an ultrahigh yield strength with large deformability, as shown for the Fe-Co-Cr-Ni-Si-B system [4]. We also present a new strategy to develop thermally stable, high-strength and deformable crystal-glass nanocomposites (Fig. 1a-d) through a thermodynamically guided alloy design approach, which mimics the mutual stabilisation principle known from symbiotic ecosystems. We realised this in form of a model Cr-Co-Ni (crystalline, ~18 nm-thick)/Ti-Zr-Nb-Hf-Cr-Co-Ni (amorphous, ~12 nm-thick) laminate composite alloy [5]. The symbiotic alloy has an ultrahigh compressive yield strength of 3.6 GPa and large homogeneous deformation of ~15 % strain at ambient temperature, with ~200 K higher crystallisation temperature compared to the original TiZrNbHf-based amorphous phase. The elemental partitioning among adjacent amorphous and crystalline phases leads to their mutual thermodynamic and mechanical stabilisation, opening up a new symbiotic approach for stable, strong and ductile materials.
These design concepts were then adapted to develop wear-resistant metals, which achieve their superior properties via in situ formation of a strong and deformable oxide nanocomposite surface during wear in an oxidative environment. This was realised for a multi-component (TiNbZr)75Ag25 alloy, by blending a TiNbZr medium-entropy alloy with Ag [6]. A ball-on-disk sliding test at maximum contact stress of 1.0 GPa revealed an alloy with low friction coefficient in air. From this tribological contact, a ~400 nm thick nanocomposite layer is formed on the crystalline alloy surface, with ~10 nm-sized Ag nanocrystals embedded in the amorphous oxide matrix (Fig. 1e). Nanopillar compression tests of the friction-induced nanocomposite reveal a yield strength of 2.4 GPa and homogeneous deformation to 20 % strain [6]. This strong and deformable oxide nanocomposite surface promotes an ultralow wear rate of the (TiNbZr)75Ag25 alloy, compared to TiNbZr or (TiNbZr)75Ag25 alloy tested in Ar atmosphere, demonstrating a pathway for designing ultra-wear resistant alloys.
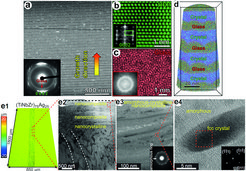
Thermal stability and oxidation resistance are also defining properties for advanced material systems, including coated components used in e.g. energy conversion applications. The MAB phase MoAlB is a promising candidate for such applications since the formation of a dense and adherent alumina scale has been reported for bulk samples. X-ray phase pure, orthorhombic MoAlB coatings were grown at 700 °C for the first time [7]. These coatings exhibit similar oxidation behaviour compared to bulk MoAlB since the oxide scale formed on MoAlB coatings after oxidation at 1200 °C for 30 min was similar to the one extrapolated for bulk MoAlB (Fig. 2), with improved oxidation kinetics than bulk Ti2AlC. High-resolution transmission electron microscopy reveals an interplanar spacing which is in excellent agreement with the lattice parameter determined by X-ray diffraction and ab initio predictions [7] as well as literature reports. In selected area electron diffraction and compositional area fraction analysis, additional, yet unidentified, Al-rich and O-rich minority phases were observed [8]. These findings are significant because the formation of 2D MBenes (2D metal borides) was observed in the vicinity of the O-rich segregations [8]. In addition to exploring the direct synthesis of 2D MBenes by magnetron sputtering, current research focuses on improving the crystal quality [9] and purity of the MoAlB coatings and heterostructures.
![Fig. 2: STEM images of close-to-stoichiometric MoAlB coating; (a) as-deposited, (b) after oxidation in ambient air at 1200 °C. The top layer corresponds to the Pt protection layer, which is deposited during the focussed ion beam (FIB) sample preparation, adapted from [7].](/4694424/original-1643028249.jpg?t=eyJ3aWR0aCI6MjQ2LCJvYmpfaWQiOjQ2OTQ0MjR9--df68bd74bee7cc2524b2a66ea84e9e0718146ae1)
References
1 Völker, B.; Du, C.; Fager, H.; Rueß, H.; Soler, R.; Kirchlechner, C.; Dehm, G.; Schneider, J.M.: Surf. Coat. Tech. 390 (2020) 125645.
2 Völker, B.; Stelzer, B.; Mráz, S.; Rueß, H.; Sahu, R.; Kirchlechner, C.; Dehm, G.; Schneider, J.M.: Mater. Des. 206 (2021) 109757.
3 Liu, C; Lu, W.; Xia, W.; Du, C.; Rao, Z.; Best, J.P.; Brinckmann, S.; Lu, J.; Gault, B.; Dehm, G.; Wu, G.; Li, Z.; Raabe, D.: submitted for publication
4 Wu, G.; Balachandran, S.; Gault, B.; Xia, W.; Liu, C.; Rao, Z.; Wei, Y.; Liu, S.; Lu, J.; Herbig, M.; Lu, W.; Dehm, G.; Li, Z.; Raabe, D.: Adv. Mater. 32 (2020) 2002619.
5 Wu, G.; Liu, C.; Brognara, A.; Ghidelli, M.; Bao, Y.; Liu, S.; Wu, X.; Xia, W.; Zhao, H.; Rao, J.; Ponge, D.; Devulapalli, V.; Lu, W.; Dehm, G.; Raabe, D.; Li, Z. Mater. Today (2021) accepted
6 Liu, C.; Li, Z.; Lu, W.; Bao, Y.; Xia, W.; Wu, X.; Zhao, H.; Gault, B.; Liu, C.; Herbig, M.; Fischer, A.; Dehm, G.; Wu, G.; Raabe, D.: Nat. Commun. 12 (2021) 5518.
7 Achenbach, J.-O.; Sahu, R.; Völker, B.; Hans, M.; Primetzhofer, D.; Miljanovic, D.J.; Scheu, C.; Schneider, J.M.: Coatings 9 (2019) 510.
8 Sahu, R.; Bogdanovski, D.; Achenbach, J.-O.; Zhang, S.; Hans, M.; Primetzhofer, D.; Schneider, J. M.; Scheu, C.: submitted for publication
9 Evertz, S.; Pöllmann, P.; Holzapfel, D.M.; Mayer, E.; Schneider, J.M.: J. Eur. Ceram. Soc. 41 (2021) 6302.