Interstitials in high entropy alloys
Interstitial alloying in high-entropy alloys (HEAs) is an important strategy for tuning and improving their mechanical properties. Strength can be increased due to interstitial solid-solution hardening, while interstitial alloying can simultaneously affect, e.g., stacking fault energies (SFEs) and thus trigger different deformation mechanisms. While this provides a variety of opportunities, it introduces at the same time a further degree of complexity. An understanding of the fundamental mechanisms on the atomic scale is therefore crucial.
For HEAs, each interstitial site displays a different local environment and thus a specific solution energy (Fig. 1). Resolving such complex local configuration dependencies is usually not accessible to experiments alone. We therefore investigated the impact of interstitials (C and N) on the equiatomic CrMnFeCoNi HEA based on first-principles calculations [1, 2]. Both, the face-centred cubic (fcc) and the hexagonal close-packed (hcp) phases of CrMnFeCoNi were modelled based on the supercell approach to evaluate SFEs. The solution energies of interstitial C and N atoms are computed for both phases. A large number of interstitial sites are investigated to elucidate the dependence on the local environments around the interstitial atoms. By scanning over various chemically different local environments, a highly realistic representation of the stability and the energetics of interstitials in CrMnFeCoNi could be obtained.
Interestingly, the distribution of the interstitial C solution energies (Fig. 1) shows a width of more than 1.5 eV, which is an order of magnitude larger than the thermal energy at relevant annealing temperatures (approximately 0.1 eV at 1000 K). This large variation of solution energies also indicates a strong dependence of them on the local environment of C. Utilizing different thermodynamic statistical treatments it is found that both, C and N, increase the SFEs [1, 2]. This is consistent with our experimental observations that interstitial C increases the stability of the fcc phase in a metastable non-equiatomic FeMnCoCrC alloy [3]. Our analysis of local correlations revealed further, that C in CrMnFeCoNi energetically prefers interstitial sites with lower local valence-electron concentration, suggesting segregation of C into Cr-rich environments, hence promoting Cr-rich carbides. Indeed, we experimentally found that Cr-rich M23C6 carbides can be found in the non-equiatomic FeMnCoCrC alloy after annealing [3]. Due to the slightly increased SFE and the trend to form Cr-rich carbides by introducing the interstitial element C, the non-equiatomic FeMnCoCrC alloy unifies all metallic strengthening mechanisms including precipitation strengthening, twinning- and transformation-induced plasticity, apart from the massive substitutional and interstitial solid solution strengthening, leading to twice the tensile strength compared to an interstitial-free HEA with similar composition, yet, at fully maintained ductility [3].
We have further extended the alloy design concept by interstitial alloying to nanostructure HEAs thereby increasing their strength and high temperature stability. This nanostructuring was explored in a model Fe19.84Mn19.84Co19.84Cr19.84Ni19.84C0.8 (at.%) iHEA processed by cold-rolling and subsequent annealing at 800 ºC and 900 ºC [4].
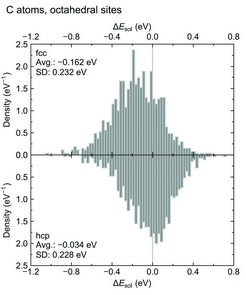
After the annealing treatment at 900 ºC the microstructure of the iHEA is characterized by recrystallized and nano-twinned regions. A deep microstructural characterized by scanning / transmission electron microscopy (S/TEM) and atom probe tomography (APT) revealed that elongated M23C6-type nanocarbides (see Fig. 2) are forming along the nanotwins, stabilizing them even up to elevated temperatures of 800 ºC. This heterogeneous microstructure leads to a significant increase in ultimate tensile strength of >1.2 GPa compared to an equiatomic FeMnCoCrNi HEA with ~700 MPa. It also provides outstanding high temperature mechanical properties resulting in yield strengths of ~650 MPa and ~300 MPa at 600 ºC and 700 ºC, respectively. The underlying mechanisms of the formation of the nanocarbides were explored by atomic resolution and in situ S/TEM. We found that nanoscale 9R structures are forming along the nanotwin boundaries before the annealing treatment. Their high dislocation content accelerates C diffusion and hence they serve as nucleation sites for the formation of the elongated M23C6-type nanocarbides. These nanophases stabilize the nanotwins enabling to overcome the trade-off between strength and thermal stability, thus qualifying these interstitially alloyed HEAs as promising pathway for the design of strong and ductile thermally stable bulk nanotwinned materials.
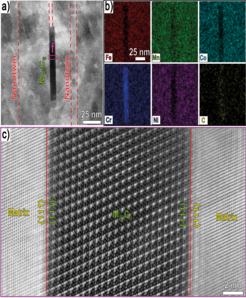
References:
- Ikeda, Y.; Tanak, I.; Neugebauer, J.; Körmann, F.: Phys. Rev. Mat. 3 (2019) 113603.
- Ikeda, Y.; Körmann, F.: J. Phase Equilib. Diffus. 42 (2021) 551.
- Li, Z.; Tasan, C. C.; Springer, H.; Gault, B.; Raabe, D.: Sci. Rep. 7 (2017) 40704.
- Lu, W.; Liebscher, C.H.; Yan, F.; Fang, X.; Li, L.; Li, J.; Guo, W.; Dehm, G.; Raabe, D.; Li, Z.: Acta Mater. 185 (2020) 218.