Advanced compositionally complex alloys
The exploration of high dimensional composition alloy spaces, where five or more alloying elements are added at near equal concentration, triggered the development of so-called high entropy (HEAs) or compositionally complex alloys (CCAs). This new design approach opened vast phase and composition spaces for the design of new materials with advanced mechanical and functional properties.
The development of alloys with tailorable strength and ductility was enabled by compositional tuning of FeMnCoCrNi alloys. By adjusting the Fe/Mn ratio it was possible to obtain single phase face centered cubic (fcc) alloys, which predominantly deform via nanotwining and dislocation plasticity, equipping them with an excellent strength-ductility synergy [1]. The key factor controlling the dominant deformation mechanism is rooted in the Mn content, which ultimately impacts the stacking fault energy as shown by density functional theory calculations. In dual-phase FeMnCoCr alloys a novel bidirectional transformation induced plasticity effect was observed, which explains the outstanding work-hardening capacity in these alloys [2]. To enhance the capabilities in mechanical properties to elevated temperatures, we also extended the alloy design concept to interstitial alloying with C in FeMnCoCrNiC alloys [3]. In this way it was possible to stabilize nanotwinned regions by the precipitation of nano-carbides up to temperatures of 800 ºC providing excellent mechanical properties for temperatures of up to 700 ºC with a tensile yield strength of ~300 MPa.
Amorphous-crystalline nanocomposite structures have been found to promote an ultrahigh yield strength with large deformability. The alloy design concept was realized in a FeCoCrNiSiB high-entropy nanocomposite [4] and Cr-Co-Ni (crystalline, ~18 nm-thick)/TiZrNbHfCrCoNi (amorphous, ~12 nm-thick) laminate composite alloy [5], respectively. Furthermore, the laminate composite alloy exhibits ~200 K higher crystallization temperature (TX> 973 K) compared to that of the original TiZrNbHf-based amorphous phase. The elemental partitioning among adjacent amorphous and crystalline phases leads to their mutual thermodynamic and mechanical stabilization, opening up a new symbiotic approach for stable, strong and ductile materials. These design concepts were then adapted to develop wear-resistant metals, which achieve superior wear resistance via in situ formation of a strong and deformable oxide crystal-glass nanocomposite surface during wear in an oxidative environment – referred to as ‘reactive wear protection’ [6]. This reactive wear protection strategy offers a pathway for designing ultra-wear resistant alloys.
In an approach to also explore the potential of CCAs for magnetic applications we combined experimental investigations and theoretical calculations to unveil an abnormal magnetic behaviour caused by addition of the nonmagnetic element Cu in face-centred-cubic FeNiCoMn-based CCAs [7]. Interestingly, upon Cu addition, the CCAs show an increase of both Curie temperature and saturation magnetization in as-cast and homogenized states. Also, the saturation magnetization of the as-cast HEAs at room temperature increases by 77 % and 177 % at a Cu content of 11 and 20 at. %, respectively, compared to the initial, Cu-free as-cast equiatomic FeNiCoMn HEA. We found that the increase in saturation magnetization of the as-cast HEAs is associated with the formation of an Fe-Co rich phase. For the homogenized alloys, the magnetic state at room temperature transforms from a paramagnetic into a ferromagnetic one upon addition of 20 at.% Cu. Both, the increase of the saturation magnetization as well as the Curie temperature could not be adequately explained by the formation of Cu enriched zones according to our atom probe tomography (APT) analysis. Our ab initio calculations suggest that Cu plays a crucial role in the stabilization of a ferromagnetic ordering of Fe, and also reveal an increase of the Curie temperature caused by the Cu, a result which agrees well with the experimental findings. The underlying mechanism behind this phenomenon lies in a combined change in unit-cell volume and chemical composition and the related energetic stabilization of the magnetic ordering upon Cu alloying as revealed by our theoretical calculations. The possibility to improve the magnetic properties of HEAs by addition of nonmagnetic elements like Cu opens a great avenue to further exploring HEAs for magnetic applications.
An alternative route to improve the magnetic properties of multicomponent alloys has been to render a massive solid solution metastable and to trigger spinodal decomposition [8]. The motivation for starting from a HEA has been to provide the chemical degrees of freedom required to tailor spinodal behaviour using multiple components. The key idea is to form Fe‐Co enriched regions which have an expanded volume (relative to a hypothetical unconstrained Fe‐Co bulk), due to coherency constraints imposed by the surrounding HEA matrix. As demonstrated by our theory and experiments, this leads to improved magnetic properties of the decomposed alloy relative to the original solid solution matrix. In a prototype magnetic FeCoNiMnCu HEA, it is shown that the modulated structures, achieved by spinodal decomposition, lead to an increase of the Curie temperature by 48 % and a simultaneous increase of magnetization by 70 % at ambient temperature as compared to the homogenized single‐phase reference alloy (Fig. 1). These findings open a pathway towards the development of advanced functional HEAs for magnetic applications.
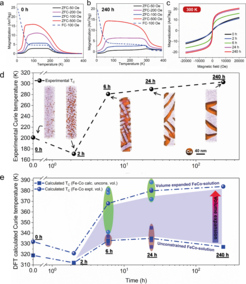
References
- Guo, W.; Su, J.; Lu, W.; Liebscher, C.H.; Kirchlechner, C.; Ikeda, Y.; Körmann, F.; Liu, X.; Xue, Y.; Dehm, G.: Acta Mater. 185 (2020) 45.
- Lu, W.; Liebscher, C.H.; Dehm, G.; Raabe, D.; Li, Z.: Adv. Mater. 30 (2018) 1804727.
- Lu, W.; Liebscher, C.H.; Yan, F.; Fang, X.; Li, L.; Li, J.; Guo, W.; Dehm, G.; Raabe, D.; Li, Z.: Acta Mater. 185 (2020) 218.
- Wu, G.; Balachandran, S.; Gault, B.; Xia, W.; Liu, C.; Rao, Z.; Wei, Y.; Liu, S.; Lu, J.; Herbig, M.; Lu, W.; Dehm, G.; Li, Z.; Raabe, D.: Adv. Mater. 32 (2020) 2002619.
- Wu, G.; Liu, C.; Brognara, A.; Ghidelli, M.; Bao, Y.; Liu, S.; Wu, X.; Xia, W.; Zhao, H.; Rao, J.; Ponge, D.; Devulapalli, V.; Lu, W.; Dehm, G.; Raabe, D.; Li, Z. Mater. Today (2021) accepted
- Liu, C.; Li, Z.; Lu, W.; Bao, Y.; Xia, W.; Wu, X.; Zhao, H.; Gault, B.; Liu, C.; Herbig, M.; Fischer, A.; Dehm, G.; Wu, G.; Raabe, D.: Nat. Commun. 12 (2021) 5518.
- Rao, Z; Dutta, B.; Körmann, F.; Ponge, D.; Li, L.; He, J.; Stephenson, L.; Schäfer, L.; Skokov, K.; Gutfleisch, O.; Raabe, D.; Li, Z.: Phys. Rev. Mat. 4 (2020) 014402.
- Rao, Z; Dutta, B.; Körmann, F.; Lu, W.; Zhou, X.; Liu, C.; da Silva, A.; Wiedwald, U.; Spasova, M.; Farle, M.; Ponge, D.; Gault; B. Neugebauer, J.; Raabe, D.; Li, Z.: Adv. Funct. Mat. 31 (2021) 2007668.