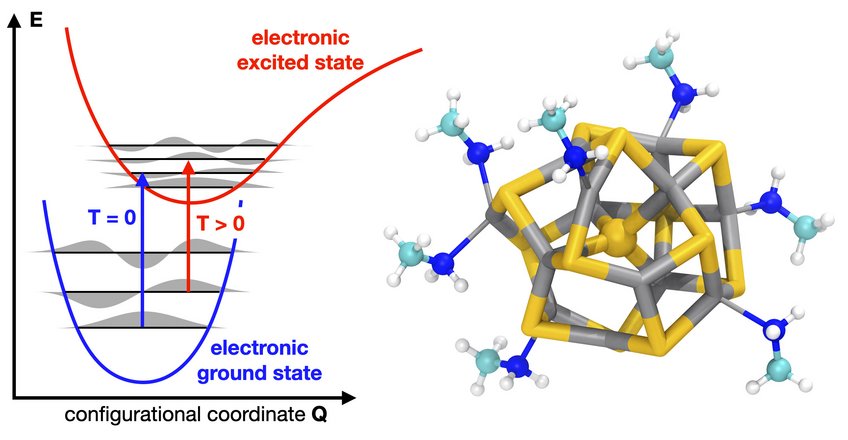
Temperature-dependent bandgap in nanoclusters
The wide tunability of the fundamental electronic bandgap by size control is a key attribute of semiconductor nanocrystals, enabling applications spanning from biomedical imaging to optoelectronic devices. At finite temperature, exciton-phonon interactions are shown to exhibit a strong impact on this fundamental property.
In materials at the border between solids and molecules, the temperature-dependence of the bandgap differs significantly from bulk materials. Due to the strong quantum confinement, excitons in nanocrystals are distributed over a spatial region encompassing only a few tens of chemical bonds, leading to a strongly enhanced exciton-phonon coupling. By occupying antibonding states, the presence of the exciton causes a pronounced weakening of the bonds and a strong exciton-induced shift of the phonon spectrum. This shift is enhanced by almost 2 orders of magnitude, compared to the bulk. As a consequence, a drastic enhancement of the temperature-dependent shift of the bandgap is observed, i. e. the photon as the probe medium itself is found to fundamentally affect the material to be studied.
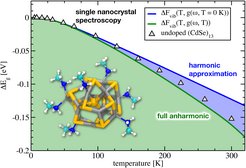
We compute the temperature-dependent band gap as the Gibbs free energy of formation for the electron-hole pair. Entropy contributions are explicitly taken into account. Using constrained density-functional molecular dynamics on the excited state potential energy surface and the principle of maximum entropy, we explore exciton-phonon coupling and compute the change in vibrational entropy associated with the creation of an exciton. Our approach allows for the first time to compute temperature-dependent bandgaps in nanoclusters with quantitative accuracy. We compare our results to spectroscopic measurements for single, monodisperse magic-sized nanoclusters.