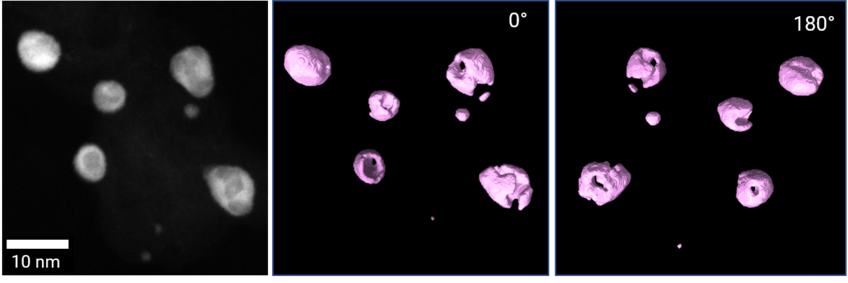
Degradation studies on fuel cells
To stop climate change, it is critical to decarbonize our energy system by replacing fossil fuels with greener alternatives. Fuel cell technology plays an important role in the transition to a world powered by renewable energy sources. This project focuses on investigating the degradation of polymer electrolyte membrane fuel cells during operation, one of the biggest issues for their widespread commercialization.
Fuel cells are devices that convert the chemical energy stored in a fuel (typically hydrogen or methanol) into electrical energy through electrochemical reactions at their electrodes. When a fuel cell is powered by hydrogen, the only by-product is water. As such, they can replace fossil-fuel engines in sectors such as shipping or trucking, or provide CO2-free electricity for our homes. In order to maximize the efficiency of fuel cells, it is essential to understand the degradation processes that occur during operation, as these degradation processes reduce the performance of the fuel cell and ultimately limit its lifetime. These degradation processes range from the diffusion/agglomeration of catalyst species to the degradation of support materials. To understand the degradation processes and their cause, fuel cells are studied before and after operation, either in real stack tests or using accelerated conditions. Different catalyst and catalyst support materials are tested to determine the relationship between fuel cells efficiency, microstructural changes and operational degradation. This is done in close cooperation with our industrial partner Freudenberg Fuel Cell e-Power Systems GmbH in the framework of the BMWk-funded project "PAULL" and the follow up project “PAULL2.0” where we team up with University Freiburg and the Technical University in Munich. The ultimate goal is to identify material systems with minimal degradation, which is necessary to achieve a long lifetime of the device.
Different degradation processes operate at different scales. Therefore, we study fuel cells at several length scales (Figure 1). Scanning electron microscopy (SEM) techniques are used to study the average composition of the electrodes and to assess their thickness and morphology. Conventional transmission electron microscopy (TEM) is used to determine the size and crystalline structure of the catalyst nanoparticles, as well as their distribution on the support material. At higher magnifications, high-resolution (scanning) TEM (HR-(S)TEM) techniques are used to locally study the chemistry and crystalline structure of the catalyst NPs, analyze their defects and determine the surface facets. Other advanced electron microscopy techniques applied in our studies include energy dispersive X-ray spectroscopy (EDS), electron energy loss spectroscopy (EELS) to probe the degree of sp2/sp3 hybridization of the support material, and electron tomography for 3D visualization and characterization of the catalyst.
We also perform correlative STEM atom probe tomography (APT) experiments. By combining the high spatial resolution of STEM with the extremely sensitive 3D chemical information obtained by APT, we can study the presence of impurities in the catalyst nanoparticles, which are known to be detrimental to their catalytic activity.
In a recently started project, we aim to use a thin film library as a model system to investigate large compositional ranges and non-equilibrium phases in a controlled manner. In particular, a thin film library of platinum (Pt) and ruthenium (Ru) prepared by combinatorial magnetron sputtering is being used to study the influence of chemical composition on structure and electrochemical performance. By combining different structural analysis tools (i.e. X-ray diffraction, electron backscatter diffraction, TEM), a length-bridging analysis of the sample microstructure can be obtained. To understand how the microstructure affects the electrochemical performance, the scanning electrochemical cell microscopy (SECCM) technique is used in collaboration with the Ruhr University Bochum. This technique allows the local catalytic activity of the sample to be measured and then correlated with its microstructure and composition.
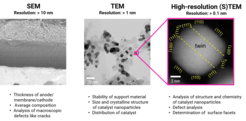
Project publications