Thermoelectric materials
Thermoelectric materials can be used to generate electricity from a heat source through the Seebeck effect, whereby a temperature difference leads to a difference in voltage for power generation. The opposite effect, known as the Peltier effect, is exploited for heating and cooling for instance. The efficiency of the conversion can be increased by introducing defects that efficiently scatter phonons, i.e. the carriers of lattice vibrations and hence heat, but do not affect much the movement of electrons so as to maintain good electrical conductivity.
In this project, we will reveal how the microstructure at the near-atomic-scale correlates with the thermoelectric performance to help guide the design of new thermoelectric materials. This will be achieved by revealing and fine-tuning the property-enhancing lattice imperfections, such as grain or phase boundaries, dislocations and secondary phases. The composition and structure of the defects in thermoelectric materials will be well characterized by a combination of electron channeling contrast imaging (ECCI) in a scanning electron microscope (SEM), atom probe tomography (APT) and scanning transmission electron microscopy (STEM). A range of thermoelectric materials are being investigated, including half- and full-Heusler as the core subjects [1-4], PbTe, AgSbTe2 and Mg3Sb2 in collaboration with internal NG-group and external groups [5, 6].
Full-Heusler: Fe2VAl
The synthesis and post-treatment of the materials play a crucial role in the development of the microstructure. In this project, we compare different synthesis routes, such as melt spinning, laser surface remelting (LSR), and spark plasma sintering (SPS) for the example of the full-Heusler compound Fe2VAl. The microstructure is then correlated locally with thermal conductivity by scanning thermal microscopy (SThM), and with electrical resistivity by a local four-point-probe technique in SEM. Figure 1 shows the remelted region after LSR. Segregation of V and N to low-angle grain boundaries and dislocations is found by APT [1, 2]. The unique microstructure within the remelted regions affects the thermal conductivity and electrical resistivity by phonon and electron scattering. TE performance of Fe2VAl is increased after LSR [2]. A more complex microstructure can be obtained by spark plasma sintering of powder. In this part of the project, we analyze Ta-doped Fe2VAl, observing a wide range of defects, such as precipitates, low- and high-angle grain boundaries, dislocation, and doping-induced point defects. We will analyze the influence of these defects on the global and local thermoelectric properties in order to understand their impact.
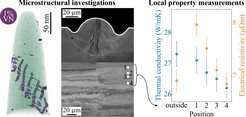
Half-Heusler: NbCoSn
The microstructure and its correlation with the thermoelectric performance of NbCo1-xPtxSn (x=0.00-0.15) were investigated. Interestingly in this series, the sample with x=0.05 (annealed, NbCoSn-Pt-AN) exhibits a striking increase in electrical conductivity compared to x=0.06 (non-annealed, NbCoSn-Pt), as shown in Figure 2. Backscattered electron images in SEM show that the post-sintering annealing leads to a tenfold increase in grain size: 0.23 ± 0.12 μm to 2.38 ± 1.65 μm. In addition, both samples have similar intragranular Pt-content and apparent segregation of Pt to grain boundaries, as revealed by APT. In situ four-point-probe technique was applied within an SEM and proves that the enrichment of Pt at grain boundaries is beneficial to the electrical conductivity [3].
Through a careful examination of the APT composition profiles crossing grain boundaries, an anti-correlation between Sn and Pt is found in the non-annealed sample. The compositional peaks of Sn and Pt are misaligned and the corresponding depletion zone only appears on one side of the grain boundary. Simulation is conducted by employing a bonding energy to show the attractive (or repulsive) interaction between Pt (or Sn) and a moving grain boundary. The good agreement between numerical and experimental results rationalizes the particular compositional profiles and proves the occurrence of the solute-drag effect on grain boundary migration. The influence of grain boundaries on the transport of charge carriers extends in a much wider microstructural region in the vicinity of grain boundaries because of fluctuations in the materials composition, which should be systematically considered in the design of thermoelectric materials targeting an optimal conversion efficiency [4].
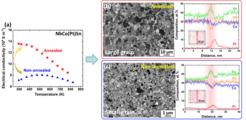