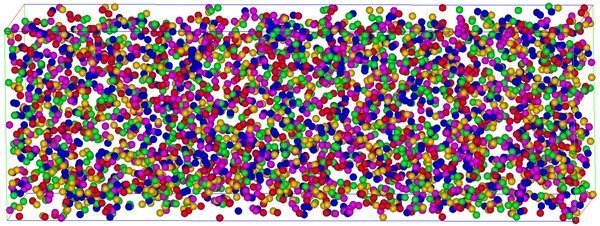
Atomic scale understanding of the TRIP-assisted dual-phase high-entropy alloys
The displacive phase transformation from face-centered cubic (FCC) γ phase to hexagonal close-packed (HCP) ε martensite and the reverse transformation (ε→γ) play a key role in enhancing the mechanical properties of FCC alloys, especially for the recently developed TRIP-assisted dual-phase HEAs. These phase transformations strongly depend on the stacking fault energy, instant temperature and stress condition during mechanical loading.
Within the statistical limits of TEM and energy dispersive spectroscopy (EDS), no indication for solute segregation and precipitation were found at interface between FCC γ and HCP ɛ phase in the dual-phase HEA. In the deformed samples, a mixed sub-structure consisting of γ nano-lamellas and twins was observed in HCP ɛ block. We also investigated the local displacive phase transformation at annealing twin boundaries in the dual-phase HEA. A systematic study is being carried out to understand the atomic structure of such annealing twin boundaries and the corresponding effect on phase transformation process.
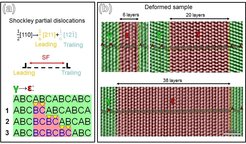
Figure 1 The mechanism of strain-induced martensitic transformation in the dual-phase high-entropy alloys upon strain loading. (a) Strain results in a separation of two Shockley partial dislocations (leading and trailing) from a perfect dislocation and then creates a stacking fault. (b) Typical high resolution HAADF-STEM images of the strain-induced ε laminates with different numbers of atomic layers in the FCC γ block after deformation.