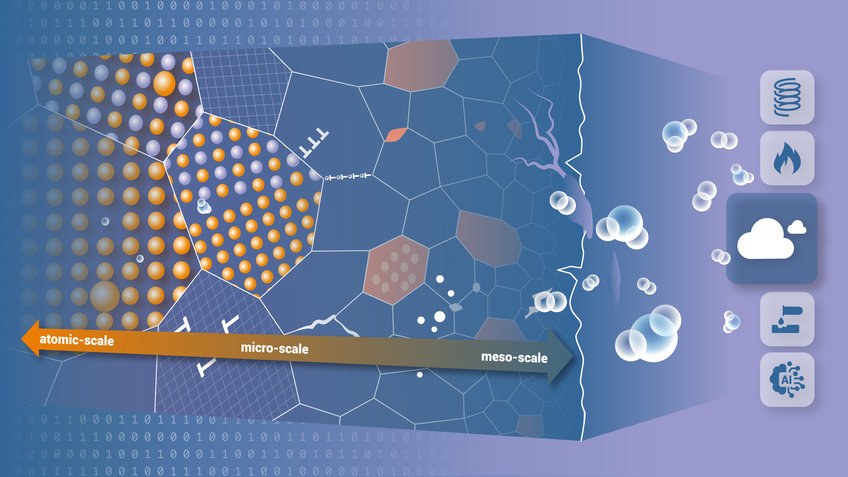
Atomic Scale Dynamics of Sustainable Materials
The research group aims to achieve sustainability by understanding and engineering the dynamic evolution of materials throughout each stage of their lifecycle, from synthesis to utilization, with a focus on energy efficiency, low carbon emissions, safety, and longevity. Employing in-situ methods for direct atomic-scale observations, we concentrate on exploring green methods of steel production during synthesis to reduce environmental impact; engineering the structural-chemical interplay in processing to enhance durability; and addressing mechanical failures, hydride formation, hydrogen embrittlement, and corrosion upon usage to boost safety and performance. Such dynamic observations down to the atomic scale, combined with theoretical interpretations, enable us to elucidate underlying mechanisms and optimize processes based on these insights, thereby refining sustainable methodologies for materials development
Aiming for net-zero carbon emissions by 2050 underscores the urgent need for innovative and transformative approaches across various sectors. This necessitates a shift towards sustainable practices throughout the entire lifecycle of metals. A thorough understanding of dynamic phenomena and the underlying mechanisms is essential to develop and implement advanced materials for a sustainable and safe future. Our focus involves meticulously observing, probing, and analyzing the dynamic interplay between structure and composition at the atomic scale. This effort aims to advance green material synthesis and microstructure engineering for outstanding performance.
Our group utilizes in-situ environmental electron microscopy to investigate the dynamics of redox reactions, specifically exploring the use of various hydrogen carriers as reducing agents in the sustainable production of green metals. This approach enables us to capture real-time chemical reactions and phase transformations within reactive gas atmospheres at the atomic scale. We focus on phase nucleation, interface propagation, and the formation mechanism of pores from mass loss. Additionally, our group explores the interplay between these phenomena and crystalline defects—dislocations, grain boundaries, phase boundaries, precipitates, and voids—to understand their collective impact on reduction. Using state-of-the-art machine learning methods, we perform automatic segmentation and object recognition for obtaining quantitative analyses of the structure and composition. By analyzing the influence of key processing parameters, such as temperature, pressure, and gas composition, on reaction kinetics, we aim to refine and optimize green reduction techniques, thereby pushing the boundaries of sustainability in the field.
The new research group also explores the dynamic response of materials, including iron, aluminum, and nickel alloys, to external stimuli such as force and heat, as well as to harsh environments, notably corrosive or hydrogen-rich atmospheres. This exploration, conducted through in-situ observations, aims to improve the performance and longevity of these alloys. We dynamically investigate phase transformations, phase separation, solute segregation, hydride formation, grain boundary migration, and the interaction of dislocations with stacking faults, grain boundaries, twins, and precipitates, due to their critical impact on enhancing material properties. By elucidating the fundamental mechanisms underpinning these phenomena at the atomic scale, we aim to contribute to the development of more durable, efficient, and environmentally friendly materials.
The group will serve as a pivotal nexus, focusing on solving problems related to the dynamics and sustainability of materials at the atomic scale. We achieve this goal by employing a broad spectrum of in-situ methods, including environmental electron microscopy, gas holders, react hubs, and microelectromechanical systems. Collaborative efforts with external research institutions, such as the Ernst-Ruska-Center in Jülich, and partnerships with internal groups in Advanced Transmission Electron Microscopy, Atom Probe Tomography, Mechanics at Chemical Interfaces, Artificial Intelligence for Materials Science, along with theoretical groups in Computational Phase Studies, Electrochemistry and Corrosion, and Computational Energy Storage Materials, will significantly enhance the capabilities of our group. Our collaborative approach drives innovation in sustainable materials science, directly contributing to a more sustainable future.