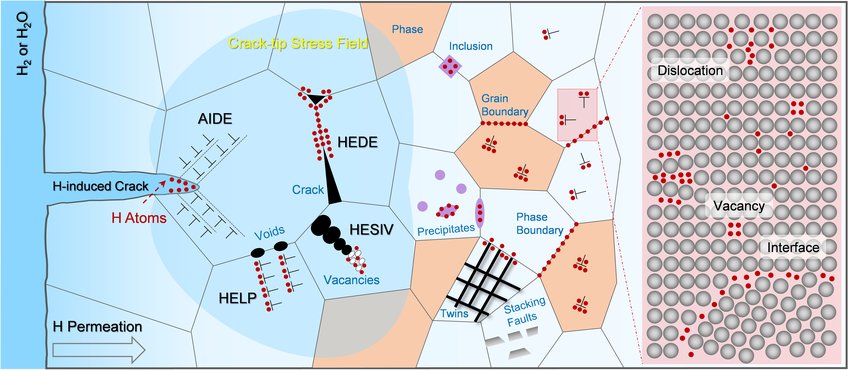
Hydrogen Embrittlement in High-Performance Alloys
Our research group focuses on one of the most dangerous yet most elusive embrittlement problems frequently observed in high-strength metallic materials: hydrogen embrittlement (HE). The mission is to understand the fundamental mechanisms of HE, as well as to use the acquired knowledge to design novel microstructure concepts with enhanced hydrogen-resistance. Ultimately, we aim to promote the development of high-performance and hydrogen-tolerant alloys that are urgently needed for the dawn of the hydrogen age.
Hydrogen (H), the most abundant element in the Universe, offers a charming sustainable energy solution that can substitute carbon-based fuels and thus reduce carbon dioxide emissions. The infrastructures and transport means required for the implementation of a H economy require affordable and mass-produced high-strength and sustainable materials. However, when the small and light H atoms make the way into a high-strength alloy (strength above ~650 MPa), the material’s load-bearing capacity is abruptly lost, a phenomenon known as H embrittlement. This embrittlement effect often occurs in an unpredictable manner, as the ingress of the ubiquitous H into a material is difficult to avoid and a H concentration of only a few parts per million by weight is often sufficient to cause catastrophic failure. Thus HE can basically threaten any industrial and societal applications in a H economy that aim to use high-performance alloys to make structural components.
Our research group firstly explores the underlying mechanisms of HE, covering three fundamental aspects in this field: H trapping and diffusion, H-defect interactions and H-induced damage evolution. Special focuses will be placed on their interplay and the association with the activation of different micromechanical models (e.g. H-enhanced decohesion (HEDE), H-enhanced localized plasticity (HELP), adsorption-induced dislocation emission (AIDE) and H-enhanced strain-induced vacancies (HESIV)). These research activities provide essential insights on the operating boundary conditions of the above HE models and their influences on the H-induced degradation of mechanical properties. The second objective of our group is to perform thermodynamics-based microstructural design in order to enhance alloys’ HE resistance at a minimum cost of their mechanical performance. Our current strategies include producing H-trapping sites to suppress H diffusion and/or forming tough microstructural ingredients to suppress H-induced crack nucleation, growth and percolation (one example shown in Fig. 1). The research is carried out by state-of-the-art characterization techniques down to the atomic level (e.g. ECCI, APT, TEM, TDS, AFM, etc.). Multi-scale simulation methods will also be combined in collaboration with simulation groups in MPIE.
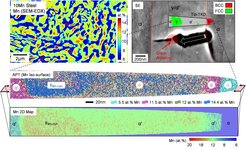