Scientific background of the seminar
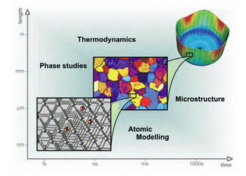
Materials exhibit a hierarchical structure. The relevant scale for the determination of mechanical properties is generally the macro scale. However, in order to understand and possibly modify the macroscopic properties of a material, an understanding of the processes occurring at smaller time and length scales is required. This is best exemplified by a deformation experiment: The formation of a macroscopically visible crack can proceed over a period of days up to several months. Complex deformations underlie the formation of this crack and it is of paramount importance how the inner structure of the material adapts to these deformations. The materials’ response to the deformation depends on a number of parameters. To start with, the details of the solidification process of the melt has a direct bearing on the microstructure and thus on the grain size distribution. The grain size distribution determines the sliding characteristics and hence significantly affects the mechanical properties of the material. Moreover, the grains in modern steels are characterized by the coexistence of several phases, whose respective transformations contribute to an increased deformability. The ductility of these materials is further determined by the mobility of dislocations, which in turn is influenced by the microstructure, as well as by the presence of solutes and precipitates.
In the course of this seminar the mechanical properties of materials will be discussed using a bottom-up-approach, starting at the atomistic scale. The above mentioned complexity underlying the mechanical properties should be disentangled by isolating the key contribution to the mechanical response. The main focus of the seminar will therefore be on the following aspects:
Methodological development aiming at multi-scale materials simulation
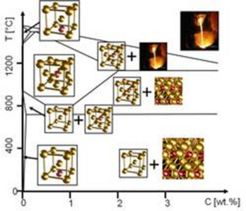
Density functional theory (DFT) is currently the method of choice for the ab initio description of solids and significant progress has been made over the last years in its algorithmic implementation. Using the calculated electron density, DFT allows to determine the energies of different structures of a given material, which is one of the fundamental preconditions for the determination of the elastic properties of a material. However, the direct application of DFT suffers from two fundamental limitations: (i) It only allows for the determination of ground state properties at T = 0K. (ii) It is computationally demanding and hence the calculation of (periodically repeated) systems containing more than about 100 atoms is already expensive, while systems containing more than 1000 atoms are currently not accessible. For the simulation of mechanical properties of materials these are considerable limitations.
It is therefore one of the aims of this seminar to discuss methods that allow for an extension in the accessible length and temperature scales. Regarding the temperature dependence, the ab-initio based treatment of of configurational entropy and magnetism will be covered. A highly topical approach in this field is the combination of ab initio data with empirical, thermodynamic tools, such as CALPHAD. The potential of such approaches for the determination of mechanical properties of materials will be one of the topics of this seminar.
The length scale issue, on the other hand, can be relieved by the development of interatomic potentials, which, however, tend to suffer from limited transferability and accuracy. In recent years interesting new approaches (e.g. bond-order potentials) to the development of such potentials have emerged, which will be discussed in detail in the course of this seminar.
Mechanical properties of bulk materials
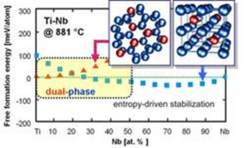
Thermodynamic stability of the bcc and the hcp phase of Ti-Nb alloys
The first requirement for the simulation of mechanical properties of materials lies in the reliable prediction of the relative stability of their structural phases. (e.g. a material in the bcc phase tends to be much softer than in the hcp phase.) The simulation of phase diagrams und phase transitions is therefore one of the central topics of this seminar, and will be closely linked to the above-described methodological section of this meeting.
Intermetallic phases are of particular importance in steels, as they can induce the formation of precipitates, which significantly affect the mechanical properties of materials, as they tend to limit the mobility of dislocations. Of particular interest in this respect are the so-called TCP (topological close-packed) phases, which should also be discussed during this seminar.
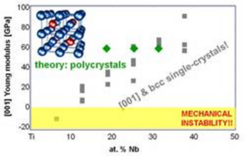
Having established the relative stability of the different phases of a particular system, the elastic tensor can be determined for the relevant phases, which can be used to predict the deformability of the material after suitable statistical averaging over the polycrystalline microstructure (homogenization). In this seminar relevant ab initio results will be considered. In particular the effect of point defects (solutes, vacancies etc.) on the materials properties shall be discussed.
Energetics and dynamics of extended defects
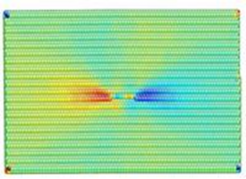
In structural materials like steels, which are characterized by a complex microstructure, the mechanical properties are determined by the physics of dislocations and grain boundaries. The plasticity of these materials is primarily determined by the mobility of these defects. Dislocation are one-dimensional lattice defects. The long-ranged strain field of these defects requires large simulation cells, which is why the above-mentioned interatomic potentials are of importance.
Over the last years fundamental progress has been made in the simulation of dislocations using both DFT and interatomic potentials. Energetic issues, such as the interaction with other defects, the splitting of the dislocation core into partials and its dependence on the stacking fault energy have been considered. Moreover, ground breaking progress has also been made recently in the simulation of dislocation mobilities. All of these developments shall be discussed in detail in the course of this seminar. This is anticipated to be the central subject of the workshop.
The microstructure and hence the mechanical properties of materials are further characterized by interfaces. The energetics of grain boundaries, as well as the accumulation of different elements at grain boundaries can already be described ab-initio (depending on the grain boundary periodicity), while the mobility of grain boundaries can be described using empirical potentials. Moreover, the on-going simulations of phase boundaries between the host matrix and inclusions will be addressed.
Failure mechanisms in materials
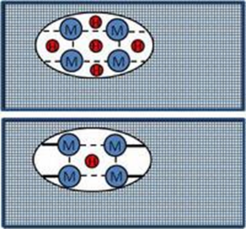
Two established mechanisms for H embrittlement: Hydride formation and decohesion
The mechanical properties of a material are limited by the onset of fracture. The fracture mechanics of metallic systems is generally characterized by extensive, dislocation-based plastic deformations (ductile fracture). The quantitative description of the core structure and mobility of dislocation is therefore of essential importance for the description of the nucleation and expansion of cracks. In particular, the development of scale transcending approaches for the simulation of fracture processes in metallic systems will be discussed in this respect.
Hydrogen embrittlement is another still controversially debated topic in steel research. The list of proposed mechanisms for the phenomenon is long (decohesion model, hydroden enhanced local plasticity, etc.). Many of these models were originally based on phenomenological observations, without detailed knowledge of the underlying atomistic processes. With the help of ab initio/atomistic methods it is now possible to systematically investigate the applicability of the different models. Such theoretical investigations provide an altogether new insight into the fundamental processes underlying the macroscopically observed embrittlement, which will be addressed in the course of this seminar.