Thermo-mechano-chemical coupling during precipitate formation in Al-based alloys
Al-based alloys are promising structural materials owing to their mechanical properties and high thermal stability complemented by their light weight. Their performance and stability, however, is largely governed by the properties of inherent second phase particles. Computationally designing technologically-relevant alloys with desired properties therefore requires a comprehensive knowledge of the underlying atomistic processes and the interplay of strain and chemistry. The aim of this project is to integrate theory and experiments in understanding the thermodynamic and kinetics aspects of thermo-mechano-chemical coupling during the precipitate formation in Al based alloys taking the example of Al-Sc and Al-Sc-Zr systems.
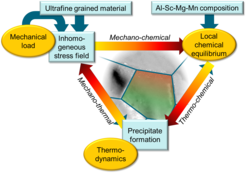
Impact of precipitate formation on solute diffusion kinetics
The kinetics of microstructure formation in an alloy is determined by the diffusion of its solutes. At the same time, the formation of nanoprecipitates generates a coherency strain field in the surrounding matrix, which influences the diffusion rate of subsequent solute atoms. This two-way chemo-mechanical coupling between the strain fields and the local chemistry (diffusion fluxes) influences the precipitation kinetics, as quantified for example, in temperature-time-transformation (ttt) diagrams.
To address this chemo-mechanical coupling, we perform DFT simulations accounting for the impact of matrix/precipitate coherency strains onto the solute diffusion [1]. The experimentally observed relationship between the size of adjacent Al3Sc particles and the diffusivities along general high-angle Al GBs serves as our benchmark. Specifically, different microstructures are produced using severe plastic deformation and post-deformation annealing experiments by our collaborators.
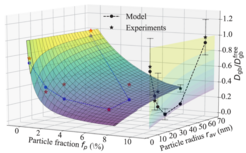
Fig. 1 shows the nonmonotonic dependence of the diffusion rates on the annealing temperature revealed by radiotracer measurements. Employing an ab initio informed modified Maxwell-Garnett model, we fully explain the observed trends. In this model, the strain field around the particle is described via Eshelby solution and its impact on the solute diffusivity in the surrounding matrix is computed iteratively within a multi-shell approach. The effects of precipitate volume fraction and the solute enrichment around the particles are accounted within a mean-field manner. In particular, the solute interfacial segregation is shown to play an important role in determining the diffusion kinetics. The study revealed that transition between coherent and incoherent precipitates explains the observed minimum in the diffusivity. The work is currently extended towards precipitation kinetics by means of kinetic Monte-Carlo simulations that take such strain effects self-consistently into account.
Finite-temperature thermodynamic properties and phase stabilities
One of our key tasks when computationally designing a material system is the ab initio determination of the Gibbs free energy, which is directly utilized for finite-temperature phase stabilities of the relevant competing phases. To this end, we often use the isobaric heat capacity as an important thermodynamic property to assess the predictive power of computational thermodynamics, as it is directly accessible from experiments and very sensitive to minor energy fluctuations. The availability of calorimetric measurements for bulk Al and the L12-ordered coherent Al3Sc particles observed in TEM studies from our experimental collaborators (PD Dr. Sergiy V. Divinski and Prof. Gerhard Wilde, Institute of Materials Physics, University of Münster, Germany) enables us to benchmark our computed free energy surface.
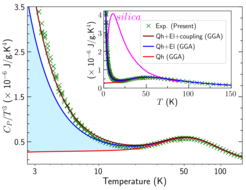
We explore the competition and coupling of vibrational and electronic degrees of freedom to the heat capacity using highly converged finite-temperature density functional theory calculations [2]. Analyzing different representations of the heat capacity, we identify and explain intriguing deviations from the Debye behavior in the low-temperature limit and in the temperature regime 30—50 K as well as the suppression of these features due to the addition of Sc (Fig. 2). As we approach melting temperatures, the finite-temperature contributions beyond the routinely employed quasiharmonic approach which include electronic excitations, anharmonicity and electron-phonon coupling become quantitatively even more significant. Taking Al3Sc as a benchmark, we demonstrate the relevance of these excitation mechanisms and their change from unary systems to alloys. This is of practical interest for the third generation of CALPHAD approaches especially in establishing thermodynamic databases for complex alloys which are challenging to synthesize in laboratory.
Microstructure formation and evolution of core-shell precipitates in Al-Sc-Zr alloys
The mechanical response and recrystallization behavior of Al-based alloys is enhanced by improving the coarsening resistance of second phase particles. A classic example which we have now better understood is the addition of Zr to Al-Sc alloys. Here, the binary Al3Sc precipitates are kinetically stabilized upon Zr segregation around the particle interfaces. Subjecting the Al-Sc-Zr alloy to varying thermo-mechanical processing conditions yields a rich microstructure decorated mainly by two distinct categories of intermetallic Al3(Sc,Zr) particles: one with homogeneously distributed Sc and Zr and the other with a Sc-rich core and a Zr-rich shell. The latter category exhibits a pronounced crystallographic anisotropy of the Zr concentration in the outer shells with a significant enrichment along the (110) and (111) oriented interfaces.
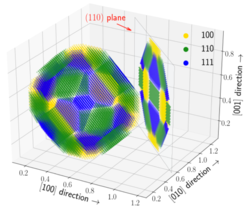
Using DFT-based calculations, we resolve this interplay between the bulk and interfacial thermodynamics resulting in the dual-phase microstructure. On the one hand, the mixing enthalpy obtained by cluster expansion predicts a homogeneous distribution of Sc and Zr as the thermodynamically stable configuration. On the other hand, the relative kinetic behavior supports the quasi-stationary core-shell structures. Our investigation in particular demonstrates the importance of preferential interfacial segregation and solute interactions thereby explaining the chemical anisotropy of core-shell structures and their subsequent evolution.
Further reading:
- Ab initio based finite-temperature thermodynamics of alloys
- Research projects in Computational Phase Studies