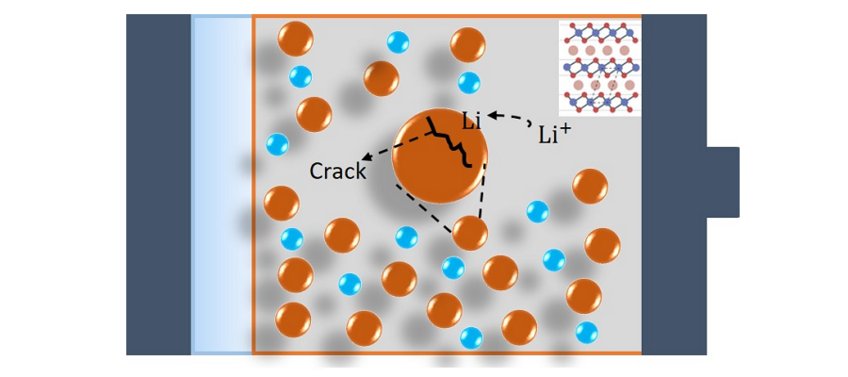
Computational Energy Storage Materials
This research group develops multi-physics and microstructure-resolved continuum models that can accurately represent electrochemical energy devices and processes under operando conditions. These models are designed to enable rationalization and improvement of the electro-chemo-mechanical performance of all-solid-state and liquid high energy density Li-ion batteries. The research activities include the construction of both physics-based and big data-driven models and their application in the studies of ion intercalation dynamics, lithium electrode heterogeneity, crystallographic defect generation, local stress responses, inter- and intra-granular crack formation, lithium dendrite growth, surface lattice reconstruction, bulk phase transition, and other electro-chemical and structural degradation mechanisms occurring during realistic battery operation.
Rechargeable Li-ion batteries represent the leading paradigm for electrochemical energy storage with high energy and power densities, and play a critical role in our life’s necessities, ranging from portable electronics to electric vehicles. However, Li-ion batteries are susceptible to electro-chemo-mechanically coupled degradation during cycling, and the root cause is complex and under debate. An operando Li-ion battery cell is a complex and dynamic system with the evolution of chemical composition, multi-scale microstructure, non-equilibrium interface reaction, and chemo-mechanical properties. The electro-chemical reactions at the interface between electrolyte and electrodes and the ion intercalation process within electrodes inevitably induce large anisotropic volumetric strain and eventually cause interfacial instability and structural degradation. Conversely, the mechanical failure strongly affects the chemical activity and even causes side reactions. In addition, the crystallographic defects, for example, dislocations, stacking faults, twin boundaries, and antiphase boundaries are commonly observed in state-of-the-art nickel-rich and Li-excess layered oxide cathodes after high voltage delithiation. These defects can regulate Li-ion intercalation, oxygen loss, transition metal dissolution, and layered-to-spinel bulk phase transformation, which play an essential role in the electro-chemical performance. Furthermore, Li dendrite formation or Li-metal penetration in solid electrolyte can cause short circuiting during plating and is one of the main forms of cell failure, whereas the correlation among dendrite formation, electronic conductivity and mechanical properties of solid electrolyte, microstructure and plastic property of Li metal, and fracture mechanics remains elusive.
The main focus of the research group is thus to develop thermodynamically consistent continuum models to establish a fundamental understanding of the multiphysics mechanisms and structural degradation inside the complex and dynamic battery cell and to correlate their effect on voltage decay and capacity loss. To this end, Butler-Volmer reaction model, Cahn-Hillard transport model, Allen-Cahn phase transformation model, crystal plasticity constitutive model, Griffith damage model, and continuum mechanics will be employed to simulate and understand the dynamic process under realistic operation conditions, in real time and on the nano- to mesoscale. The group also actively collaborates with experimental and ab-initio research groups, both in terms of mechanistic understanding of experimental results, assisting the microstructure design of high-performance electrodes, and by directly constructing the geometrical and physical models from experimental and atomic simulation data, such as electron microscopy techniques, operando diffraction analysis, GITT experiments, solid-state nuclear magnetic resonance spectroscopy, and nano-indentation experiments.
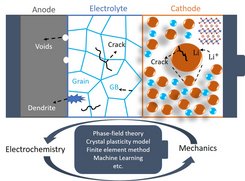