Understanding Hydrogen adsorption on Pt Under Realistic Electrochemcial Conditions
We use ab initio based molecular dynamics simulations to understand the thermodynamic driving forces triggering electrochemical reactions that involve hydrogen adsorption on Pt electrodes to gain fundamental understanding of processes at solid/liquid interfaces and aid the design of better electrocatalysts.
The hydrogen evolution reaction (HER), which is the cathodic reaction in electrochemical water splitting, is one of the most intensively studied electrochemical reactions because of its importance to hydrogen based economy/green economy. The strength of hydrogen adsorption on transition metal/water interface is known to determine its catalytic activity for the HER. Gaining a microscopic understanding of the fundamental nature of hydrogen adsorption on such interfaces will aid improving the catalytic activity of existing catalysts and/or the design of novel efficient catalysts for this reaction. Obtaining such insights from experiments alone is difficul, therefore theoretical/computational insights are highly welcome. Considering that adsorbate bonding at such electrochemical interfaces is determined by the electronic structure at the interface, electrostatics, and the dynamic nature of the electrolyte/solvent, computational models that include all these effects are required for the accurate modelling of such systems.
![Fig.1: Work function (blue) and time-averaged electrode potential (green) as function of qH coverage on Pt(111) with respect to the DFT computed work function of the clean Pt(111) surface (5.50 eV). For comparison, the experimentally measured work function [2] (black line) and electrode potential [3] (black crosses) are included. The critical H concentration is predicted to lie the yellow hashed area and is extrapolated as indicated by the dashed green lines. Representative surface and interface structures at select coverages are shown as insets with the grey, red, green, and white spheres representing Pt, O belonging to water molecules, O belonging to a solvated hydronium ion, and H atoms respectively.](/4730817/original-1645386061.jpg?t=eyJ3aWR0aCI6MjQ2LCJvYmpfaWQiOjQ3MzA4MTd9--b67b78988239c311347ad80ae376ab8808f87ad7)
To this end, we performed density functional theory based molecular dynamics (DFT-MD) simulations of hydrogen covered Pt(111)/H2O interfaces. We chose Pt, since the Pt/H2O is one of the best studied electrochemical interfaces due to its high catalytic activity for the HER [1]. We compute the thermodynamically averaged interfacial work function (electrode potential) for interfaces with differing hydrogen adsorbate coverages and compared them to data obtained from experimental cyclic Voltammetry [Fig. 1]. We find that the co-adsorption of H2O molecules on these surfaces lowers its work function and stabilizes the interface at experimentally relevant electrode potentials. On increasing H adsorption however, the decrease in the number of chemisorbed H2O molecules and the subsequent increase of the work function destabilizes the interface at high H coverages. Our results explain, for the first time, why there is a maximum critical hydrogen coverage of 0.66 monolayers observed experimentally [3] and the driving force for electrochemical desorption of hydrogen from the surface at potentials which correspond to the onset of hydrogen evolution.
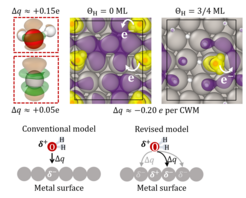
Analysis of the electronic charge density rearrangement at the Pt/H2O interface reveals that electronic charge is not transferred from an adsorbed H2O molecule to the Pt atom on top of which it is found, as assumed in the conventional bonding model, but to the Pt atoms surrounding it [Fig. 2]. This makes H2O chemisorption sensitive to co-adsorbates on the surface and explains the decrease in H2O chemisorption with increasing hydrogen coverage.
Presently, the focus is on extending these studies to understand the thermodynamic stabilities of the hydrogen adsorbates at various adsorption sites and the corresponding structural and spectroscopic signatures under realistic electrochemical and ultra-high vacuum (UHV) conditions, in collaboration with experimental partners. We furthermore consider also more open Pt surfaces like Pt(100) to understand how the observed effects are influenced by the surface geometry.
References:
[1] J.N. Hansen, H. Prats, K.K. Toudahl, N.M. Secher, K. Chan, J. Kibsgaard, I. Chorkendorff, Is There Anything Better than Pt for HER?, ACS Energy Lett. 2021, 6, 1175–1180 (2021)
[2] P.R. Norton, J.W. Goodale, Hydrogen on Pt(111) studied by dynamic measurements of the work function, Solid State Commun. 31, 223-227 (1979)
[3] N.M. Marković, B.N. Grgur, P.N. Ross, Temperature-Dependent Hydrogen Electrochemistry on Platinum Low-Index Single-Crystal Surfaces in Acid Solutions, J. Phys. Chem. B 101, 5405–5413 (1997)