Atomic Insights into Complex Materials – High-end Atom Probe Tomography and the Story behind it
Hi,
I’m Baptiste. I lead two research groups at the Max-Planck-Institut für Eisenforschung (MPIE). One is focused on atom probe tomography, and the other… well also on atom probe tomography but targeting specifically hydrogen and is funded by a Consolidator grant of the European Research Council that I was awarded in 2018. In short, in the group we develop and use this microscopy and microanalysis technique to investigate the composition of materials at the near-atomic scale to better understand how the nanoscale structure of a material influences its behaviour– with an aim to use this knowledge to guide the design of higher performing or more enduring materials.
I was invited to guest edit this newsletter as I was awarded the Leibniz Prize 2020 by the German Research Foundation.
Receiving the Leibniz Prize has been the greatest honour and, well, the greatest surprise. Thinking of my job, what comes to mind is a famous quote from Gary Lineker[1], who, in the 1990s, said “Football is a simple game, twenty-two men chase a ball for 90 minutes and at the end, the Germans always win”. I see research as a team sport. In the end, women and men of different nationalities and disciplines together chase understanding of experimental and theoretical data, and at the end, Science wins. At the moment, Germany also wins often, thanks to the high level of support that we are getting – Germany is in the top 10 worldwide in terms of R&D expenditure per capita.
I love my job here at the MPIE. Our key strengths lie in the blend of expertise, in our collaborative spirits and the very talented and smart early-career scientists with whom we work. Atom probe has become part of, or central to a wide range of studies on material. I selected and will discuss just some amongst the very exciting work with which I had the chance to be involved.
[1] Gary Lineker is a famous English football player of the 1980s.
The Past...
The Prize was awarded to me for my frontier work in atom probe tomography (APT) and the research that the technique enables. APT is unlike any other microscopy and microanalysis technique. In APT we peel off individual atoms from a specimen, measure their mass, attribute an elemental nature, e.g. is it Fe or Al or C or W, and then build a digital three-dimensional point cloud to reposition each of these atoms as close as possible to where they were originally located inside the material. From within this virtual 3D map, we can then dig and retrieve information. Sometimes this is done manually, sometimes through applying more advanced data extraction, including machine learning or other artificial intelligence techniques. The information gleaned from the data is mostly the local composition of the different microstructural features that are contained within the specimen. Sometimes, imperfect structural information can also be retrieved and used to help guide the reconstruction process or e.g. identify phases. APT has found application in physical metallurgy in semiconductors, both for academic and industrial research (Samsung in South Korea has more than half a dozen instruments now). The technique is gaining momentum in fields such as geology and, maybe, in the future, in the biological sciences.I have been fortunate, over the course of my research career to work on almost all aspects of atom probe science. The Prize was given to me at least in part for what I initiated during my PhD, which was concerned with implementing an ultrafast laser source on an atom probe and deciphering the physics of the field evaporation process. The interaction between a coherent light pulse lasting only tens of femtoseconds and the APT specimen, a sharp needle with dimensions that are only tens of nanometres and hence smaller than the light’s wavelength, is indeed non-trivial. So non-trivial that most of the interpretation of the data within my PhD thesis was proven wrong in the following years. Yet, my pioneering work was a great enabler in the development of the technique, and pulse-laser sources are now implemented on nearly all commercial APT instruments. In the following years, as a postdoctoral scientist, I worked also on improving the approaches to build the tomographic reconstruction, and working on quantifying more precisely the spatial performance of the technique.Another important aspect for me was to start using the technique for materials characterisation – an opportunity to start using my knowledge in materials science, but also to develop some more understanding of how materials behave – I worked on Al-alloys, steel, zirconium alloys, semiconductors amongst others. Over 2010–2012, alongside my colleagues at The University of Sydney, we wrote a book on atom probe, which was the first one to be published for almost a decade. For me, it was a tremendous experience, allowing me to gather solid knowledge on all aspects of the technique.Since joining the MPIE in 2016, I cannot think of a single aspect of this previous experience that has not been useful. I have had a chance to renew my interests in:
- Instrument development via the Laplace project (see separate section)
- Data mining, in part via our involvement in the BIGMAX network that links Max-Planck-Institutes interested in exploring machine-learning approaches to help design new materials
- Theory of field evaporation and field ionisation with the development of the analytical-field-ion microscope
- And very many materials science projects, with an emphasis on understanding the fundamental processes that take place in complex, engineering materials, especially over the course of their use in service, insights that are necessary to guide design of future materials with enhanced lifetime and improved sustainability.
Complementing APT with TEM and complementing TEM with APT
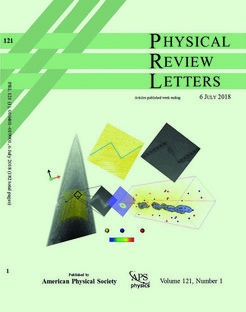
Since before I joined, one of the key areas that the MPIE had been pushing was complementing APT with transmission electron microscopy (TEM) and complementing TEM with APT, in part through the work of Dr. Michael Herbig. This, in part, motivated the Laplace Project, but also is at the core of my work with Prof. Christina Scheu’s and Dr. Christian Liebscher’s groups. Our collaboration led to having atom probe tomography and high-resolution transmission electron microscopy on the cover of Physical Review Letter – one of the most prestigious scientific journals (see Fig. 1). This work was focused on understanding the behaviour of minor impurities in silicon, which are known to affect the performance of solar cells. We combined information on the intimate structure of grain boundaries with their local composition from atom probe. With the aid of atomistic simulations performed by Dr. Liverios Lymperakis from the Computational Materials Design Department, we concluded on the critical role of strain on the segregation of impurities at specific locations along the grain boundary facets or their junctions [1].
With Christina, we keep working closely on a range of functional materials, often also in collaboration with Dr. Olga Kasian (formerly department “Interface Chemistry and Surface Engineering”, now at the Helmholtz Zentrum Berlin). We combine APT and TEM for compositional and structural analyses, and use that information to understand catalytic or electrocatalytic processes, with an aim to help design materials with better performance and enhanced longevity. Materials of interest here find application in the hydrogen economy, for water splitting for instance [2; 3], or nanomaterials, which are frontier applications of APT [4; 5].
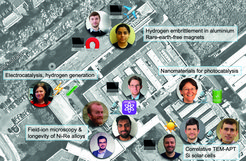
Bridge to atomistic simulations
A key strength of APT is its compatibility in scale with atomistic simulations. Let me here mention joint projects with Drs. Tilmann Hickel and Christoph Freysoldt from the Computational Materials Design department. With Tilmann, we work closely on hydrogen embrittlement in aluminium, which is at the core of the ERC-funded project of Dr. Chakraborty (see section "Scientists at the MPIE"). We have used insights from APT to guide ab initio simulations to better understand the influence that hydrogen can have on grain boundaries in alloys that find application in the aerospace industry. We also work on trying to better understand the influence of the microstructure on the magnetic properties of rare-earth free magnets.
![Fig. 3: a Field ion micrograph in which each bright spot is an individual atom. b Close-up on a specific set of atomic planes, the yellow lines indicate that an extra half-plane is present: a dislocation induced by the plastic deformation. Two bright spots appear at the defect. c Results from density-functional theory reveal that the imaged bright atoms correspond to Re-atoms [6].](/4319856/original-1590499399.jpg?t=eyJ3aWR0aCI6MjQ2LCJvYmpfaWQiOjQzMTk4NTZ9--de076d5446f7747263fd1a65697a63714bd79ede)
With Christoph, we worked on explaining the contrast between atoms of different species in field-ion microscopy by using density-functional theory under intense electrostatic-field conditions. Drs. Leigh Stephenson & Shyam Katnagallu started exploring the possibility of re-visiting field-ion microscopy and combining it with time-of-flight mass spectrometry [6]. We designed an experiment using a binary Ni-Re alloy that had been subject to creep deformation at high temperature, and is core to our activity in superalloys, led by Dr. Paraskevas Kontis. They visualised bright atoms specifically at crystal defects. Christoph’s input allowed us to interpret the contrast, which was then verified by experiments (see Fig. 3). This feedback loop between experiments and theory was crucial to really understand the results for the first time that Re was directly observed to be segregating to dislocations in this alloy system. This helped in rationalising a 40-year-long engineering observation that Re increases the creep lifetime of Ni-based superalloys, and gave evidence of a similar effect in complex alloys by combining electron microscopy, atom probe and atomistic phase-field simulations [7].
The Future
One could wonder what is the next step in all this? What are the next frontiers? To me, it is deploying the strength of atom probe to the analysis of liquids, liquid-solid interfaces and soft matter. Since the 1950s, several scattered investigations of biological materials by atom probe have been reported, but without a sustained effort that would have enabled true breakthroughs. In parallel, the development of cryo-TEM has initiated a revolution in the biological sciences, enabling to image proteins and viruses at an unprecedented resolution. Atom probe could, here again, provide precious and unique insights – an example is the presence of metallic species suspected to be involved in the Alzheimer disease. A few years ago, we started looking at such proteins in a collaboration with a group at Heinrich Heine University Düsseldorf. The grant from the Leibniz Prize will allow to complete this research, via cryo-specimen preparation, transport and analysis of frozen liquids, which can be used as carriers of such biological materials.
I really want to thank Prof. Dierk Raabe for opening the door for me to come back to research after spending some years at Elsevier, and do what I do best.
Un grand Dankeschön.
I want to say how grateful I am for the support I get from Sandy, my wife, who’s been putting up with me for 20 years and with whom we have lived in 5 countries, and our lovely daughter Violette. To quote a great Canadian poet: "everything I do, I do it for you".