Permanent Magnets – Research for Sustainability
The RE element neodymium (Nd) is increasingly often used in high performance magnets that are employed for many applications such as electrical vehicles and wind turbines. For example, 700 kg sintered Nd magnet is needed for a wind turbine with a 1 MW power capacity. The element dysprosium (Dy) improves Nd magnets by enhancing their coercive force and resistance to demagnetization. Both elements suffer, however, from mining and processing conditions that cause a large environmental footprint. Even more severe is their supply risk (Fig. 1), since over 90 % of the RE elements worldwide are currently produced by a single country (China).
![Fig. 1: Supply risk of RE elements plotted versus their importance for clean energy applications. Adapted from [1].](/4185387/original-1579794815.jpg?t=eyJ3aWR0aCI6MjQ2LCJvYmpfaWQiOjQxODUzODd9--0be33169ccadabf599058a0a4af17a2aa6dc3138)
Fig. 1: Supply risk of RE elements plotted versus their importance for clean energy applications. Adapted from [1].
Due to the criticality for future technology, one needs to find alternative hard-magnetic materials that are based on more abundant elements. According to Fig. 1, Cerium (Ce) is a candidate with a much lower supply risk, but its potential for clean energy applications is still largely unexplored. Ce-based hard magnets are, therefore, in the focus of our research. Although in particular the CeFe11Ti phase has – due to its excellent magnetic properties – a great potential to replace Nd-based magnets, there are also severe challenges. Most critical is the formation of magnetically detrimental Laves phases, such as CeFe2. They tend to dominate the microstructure, retarding the crystallization of the desired hard magnetic phase.
We are using sophisticated ab initio based methods to calculate finite temperature phase stabilities. Employing state-of-the-art approaches for vibrational, electronic, and magnetic entropy contributions, the Helmholtz free energy is calculated for the desired hard-magnetic ternary phase and all relevant binary phases, as shown in Fig. 2. Consequently, the thermodynamic stability of CeFe11Ti as compared to a set of competing phases can be accurately evaluated [2]. The ab initio calculations predict a critical temperature below which a decomposition to Laves phases occurs, serving as a guideline for a thermodynamic processing.
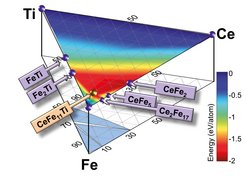
Fig. 2: Heat map of the calculated free energies of formation for the Ce-Fe-Ti phases at T=1500 K.
In order to tailor these phase stabilities, we have considered the effect of quaternary additions. To explore Ce-Fe-Ti-X alloys for all 3d and 4d transition elements X, we developed an efficient screening method and were able to demonstrate that alloying elements such as Zn can decrease the critical temperature substantially, making the material system more attractive for applications. The study therewith demonstrates that the substitution of established hard magnets by more sustainable material systems, requires a careful analysis not only of the magnetic performance, but also of the thermodynamic stability.
References:
[1] D. Bauer et al.: Technical report, U.S. Department of Energy (2011).
[2] H. I. Sözen et al.: Phys. Rev. Mater. 3 (2019) 084407.
Authors: H. Sözen, T. Hickel (CM)