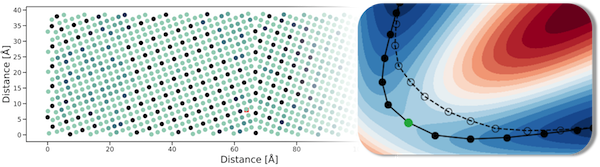
Thermodynamics and Kinetics of Defects
Research in the Thermodynamics and Kinetics of Defects group focuses on the interplay and co-evolution of chemical and structural defects in structural metals to better understand and predict the role these defects play in macroscopic material properties.
This research group is no longer active!
Liam Huber has moved back to Canada and takes a break from Academia.
At thermodynamic equilibrium a metal contains only isolated point defects, e.g. vacancies and isolated solute atoms. In reality however, this state is never reached and real materials are rich with a wide variety of defects, including dislocations, grain boundaries, and precipitates of various sizes. These defects have a profound influence on the final material properties, e.g. the famous Hall-Petch relationship relating the average grain size to the yield strength. In turn, the behaviour of structural defects is often strongly influenced by chemical defects which are either introduced intentionally through alloying, or unintentionally through exposure to the environment. Because chemical and structural defects often interact strongly, the local concentration of solute atoms in the neighbourhood of a structural defect often far exceeds the bulk concentration. In this way even very small amounts of an alloying element can play an out-sized role in final material properties.
Simulating the interaction and co-evolution of structural and chemical defects at the atomic scale presents two significant challenges. The first is to bring together different length scales: realistic structural defects often require large simulation domains, but a high degree of accuracy is still needed for representing the very local interaction of each solute atom with these defects. The second is the broad issue of sufficient sampling, whether in configurational space -- a single grain boundary, for instance, has five dimensions which must be specified, and the distribution of solutes at such a grain boundary is combinatorically complex -- or in time -- obtaining sufficiently accurate thermodynamic averages becomes difficult as temperature increase, and as temperatures decrease observing various kinetic effects directly can become difficult or impossible.
To address these issues, we bring together several approaches:
- The application and development of physical models which go beyond brute-force to uncover thermodynamic states and kinetic rates.
- Multi-scale methodology that brings together the strongest features of simulation techniques at different scales and levels of accuracy/computational cost.
- The use of machine learning to develop computationally efficient 'surrogate models' to help tackle the combinatorically vast space of possible structural-chemical configurations.
- Ongoing development of a computational platform (pyiron) for rapid prototyping of new algorithms and models.
These approaches do not exist in a vacuum, but are driven by the need to study particular material systems which are relevant to materials engineering and design. These needs are identified in close collaboration with our experimental colleagues, and current work has a special focus on light-weight Al- and Mg-based alloys.