Planar defects in off-stoichiometric, Nb-rich NbFe2 Laves phase
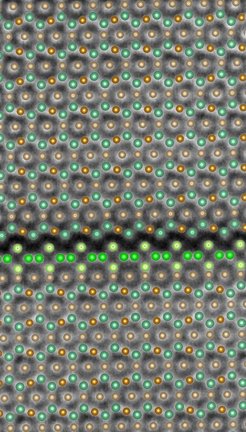
In this project, we highlight an example of binary intermetallic alloys that show such phenomena: Groups of Advanced Transmission Electron Microscopy (Christian Liebscher) and Intermetallic Materials (Frank Stein) have observed novel atomic structures connected to planar stacking faults in phase-pure samples of the hexagonal Laves phase NbFe2 . These faults substantially change phase concentration and therewith the mechanical performance of a phase, which is important for precipitation hardening in high-temperature steel applications [1-3]. The significant increase in the defect density of the Laves phase NbFe2 was observed with scanning transmission electron microscopy (STEM) in samples with 35 at.% Nb, while stoichiometric samples (33 at.% Nb) showed only isolated dislocations. Besides antisite occupation, various types of planar faults appear. We observed numerous extended planar defects parallel to the basal plane of the hexagonal structure, introducing Nb-enriched layers of various thicknesses, as well as a high number of confined basal and pyramidal planar faults. All types of observed basal and pyramidal faults contain structural motifs, which are characteristic for the crystal structure of the μ phase Nb6Fe7 (46 at.% Nb) in the same binary system. Interestingly, however, the stacking of the μ phase is not fulfilled in the atomic layers next to the defects, despite the increased Nb content. One of the variations in the stacking sequence is the omission of an Fe-layer, so-called Kagome layer, as shown in Fig. 1.
We used ab initio calculations to determine the formation energies of these planar defects. A prerequisite was to find the exact crystallographic structure underlying the experimental STEM images, since the restriction to a single projection is still consistent with multiple translational variants perpendicular to the image plane. Therefore, the generalized stacking fault energy (SFE) surface was determined by ab initio calculations (Fig. 2). The energy of the most stable crystallographic Nb-enriched defect structures was then compared with a phase separation of the off-stoichiometric composition into a perfect Laves and a perfect μ phase. While the defects turned out to be not stable in equilibrium conditions, some of them become energetically favourable as compared to a phase separation that involves a laterally strained μ phase. This explains why a nanoprecipitation of μ phase is less likely than the observed extended defects.
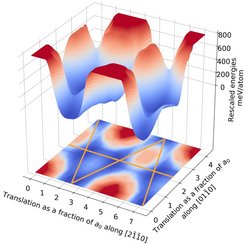