Non-radiative recombination in optoelectronic devices
Optoelectronic devices allow for the direct conversion of light and electrical energy. The performance of such optoelectronic devices is limited by losses due to non-radiative recombination. We have looked at the solar cell material Cu(In,Ga)Se2. We identified not only an effective recombination pathway at high Ga contents involving configuration changes of Ga or In anti-sites, but also extended the charge corrections to the computation of configuration coordinate diagrams.
Optoelectronic devices allow for the direct conversion of light and electrical energy. The underlying materials are semiconductors with a band gap lying in the range of the photon energy of visible light (around 2-3 eV). In light-emitting diodes (LEDs), electrons in the conduction band (states above the gap) and holes in the valence band (states below the gap) recombine radiatively, i.e., the emit light. In solar cells, the reverse process happens: by absorption of photons (light), an electron is lifted from the valence band to the conduction band, leaving behind a hole in the former.
The performance of such optoelectronic devices is limited by losses due to other, non-radiative recombination processes. One important example is “Shockley-Read-Hall” recombination, named after the scientists that first proposed a plausible model. The key role in this model is a defect with an electronic level in the band gap that is strongly coupled to the atomic vibrations (i.e. phonons). Coupling here means that the energetic position of the electronic level varies with the atomic configuration. In one such (displaced) configuration, the level could be close to the conduction band and capture an electron. In another one, it could be close to the valence band, and thus capture a hole (by releasing the electron to it). The electronic energy associated with the difference between the conduction band and the valence band is converted to vibrations, i.e., heat. Fortunately, not all defects occuring in semiconductors show this strong coupling. For optimizing performance, on the other hand, it is important to identify the problematic ones that do, and develop processing strategies to avoid them. Theoretical simulations can help to identify relevant cases by studying the electronic structure in displaced configurations. This is typically depicted in “configuration coordinate diagrams”, where crossing lines indicate a degeneracy of the defect level with the valence band (for holes) or conduction band (for electrons). To reach the crossings from the minima of the curves requires an activation energy, which largely determines the kinetics of the process.
![Configuration coordinate diagram for recombination via an DX-like configuration of the GaCu antisite in CuGaSe2, an endmember of the Cu(In,Ga)Se2 (“CIGS”) alloy system for photovoltaic applications. Adapted from [1].](/5037067/original-1736269311.jpg?t=eyJ3aWR0aCI6MjQ2LCJvYmpfaWQiOjUwMzcwNjd9--cb64eb6608676170877d4ba30d689ce568327536)
With the aim to develop methods and insights transferable to other thermally activated electron transfer processes simulations, we have looked at the solar cell material Cu(In,Ga)Se2. We identified an effective recombination pathway at high Ga contents involving configuration changes of Ga or In anti-sites [1]. This underlines the important role of metastable configurations for the recombination pathways, that was also found in other systems [2].
In this context, we also extended the charge corrections to the computation of configuration coordinate diagrams where the change in ionic screening is decoupled from the actual system charge. This has been made available in the latest version of the sxdefectalign tool [3].
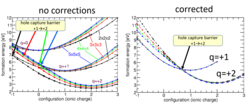