Development of ab initio simulation techniques to investigate surfaces under realistic environmental conditions
Let us take the example of corrosion – while it is known that, at the fundamental scale, a metal reacts with species from the environment and transforms into an oxidised form, the reactive intermediates in the occurring multi-step electrochemical reaction and their exact place within the reaction chain continues to be a subject of heavy debate. Gaining insight into the microscopic processes occurring at a solid/liquid interface presents a challenge to both experimental measurements and theoretical modelling. This is due to the involvement of different classes of material systems on the solid side of the interface (metals or semiconductors), which may impose different requirements on investigational approaches. Electrolytes are, on the other hand, probably most challenging regarding their dynamics, configurational diversity and tendency of aqueous electrolytes to form H-bonding networks. The electric fields evolving at the interface couple to and influence the relevant surface processes, while the involved time and length scales present a challenge to any form of theoretical modelling based on quantum mechanical methods.
The posed questions can be systematically addressed by determining the thermodynamics and kinetics of the surface, as well as of the ions in solution and in the oxidised layer. In this context, ab initio electronic structure calculations can offer a complementary view to experimental investigations, in analogy to what is common and very successful practice in the realm of surface science. There, a key to success are surface phase diagrams, which identify the thermodynamically preferred surface termination as a function of chemical potentials. Such diagrams rely on an accurate determination of free energies at finite temperatures and are constructed by coupling density-functional theory calculations to thermo-dynamic considerations, which opens a route to explore and understand the impact the environment has on a system while retaining the predictive power of the first-principles method.
The availability of surface phase diagrams allows reducing the multitude of fast processes and the identification of the thermodynamically stable surface phases, as well as the sets of conditions which define the boundaries for their stability. Thus, such diagrams provide the basis for determining the critical reactions taking place at the interface and could, for example, help us to understand what impact hydrogen might have on the protective Zn layer used on steels or how a sour gas environment changes the properties of a steel surface. Extending such diagrams to electrochemical conditions, by accounting for changes in pH and potential conditions (as they determine and characterise the state of an electrochemical system) appears, therefore, highly desirable.
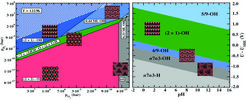
Surface phase diagram for a ZnO(0001)-Zn surface in equilibrium with a humid oxygen atmosphere at T=950ºC (left) and an electrochemical environment at T=300ºC (right). Geometries of the stable surface structures are shown as insets. Equilibrium conditions under which water would condensate on the surface are indicated by a white hashed area in the left picture.
Recently a method which seamlessly and quite naturally links density functional theory calculations to such experimental observables as the pH-scale and the electrode potential was developed in the group of Mira Todorova at the Computational Materials Design department of the MPIE. The approach is based on the formation energies of ions in solution, which are utilised in a thermodynamic context. It is inspired by the observation that, from a formal point of view, ions in solution and charged defects in semiconductors – the fundamental building blocks of theoretical and modelling concepts in semiconductor defect chemistry and electrochemistry – are equivalent, which makes it possible to unify and “translate” concepts of these two fields. Employing this approach provides surprising new in-sight into apparently ”old” problems such as water stability. It also opens, for example, new routes to the construction electrochemical phase (Pourbaix) diagrams, allowing also for the consideration of surface phases or nanostructures, which effectively ex-tends the concept of surface phase diagrams to the consideration of electrochemical conditions.
Author: Mira Todorova