Complex Interplay of Grain Boundary Structure and Mechanics
Grain and phase boundaries are omnipresent in materials. It is commonly known that increasing the boundary density (i.e. decreasing the crystallite size) results in pronounced hardening, empirically described via the Hall-Petch relation. However, the impact of the local slip geometry, the atomistic grain boundary structure and chemistry on the break-through stress of dislocations is quantitatively unknown.
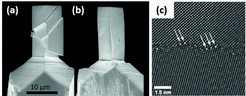
Fig. 1: SEM image of a (a) single and (b) bi-crystalline micro compression sample. (c) Asymmetric high angle grain boundary in copper with silver atoms (white arrows) segregated at the facetted grain boundary. Reference: Peter, N. et al: J. Mater. Res., 32 (5), 968-982 (2017). Reprinted with permission.
To unravel the fundamental question of the underlying slip transfer mechanism and establish general material models a research strategy comprising small scale in situ deformation in the scanning electron microscope (SEM) and at Laue microdiffraction (μLaue) synchrotron beamlines together with advanced microstructure characterization in the transmission electron microscope (TEM), is currently applied. Testing the mechanical response of single and bicrystalline submicron sized samples permits to study the mechanical impact of one individual grain boundary without being blurred by the surrounding bulk (see Fig. 1 a, b). But the testing protocol allows for more than just being a mechanical microscope. Due to the in situ capabilities of μLaue and SEM based techniques, the number and type of stored and transmitted dislocations can be measured while monitoring the required stresses for deformation – giving a comprehensive picture of the collective transmission properties of a grain boundary. The additional use of TEM based techniques does not only give insights on the interaction of one individual dislocation with a grain boundary, but can also be used to establish the atomic structure and chemistry of the grain boundary before and eventually after deformation.
First results show that the dislocation transmission stress through coherent copper twin boundaries can be as low as 17 MPa, which is currently supported by atomistic modelling made by the MPIE’s Computational Materials Design department. Nevertheless, for pure general high angle grain boundaries the definition of a breakthrough stress might not be a suitable strategy to describe the slip transfer properties of a grain boundary, as in this case the slip transfer behaviour is highly strain rate dependent. These characteristics can be vastly different in the case of alloyed materials, where segregation induced changes in grain boundary chemistry occur: An indication of faceting, either caused or stabilized by solutes was recently observed by high resolution scanning TEM in Ag alloyed Cu samples (see Fig. 1c). These changes in atomic structure and chemistry can result in local stress concentrations and / or a hindered, non-conservative motion of dislocations in the grain boundary plane. The connection of both, the atomic structure of individual grain boundaries and their interaction behaviour with dislocations is focus of our current investigations.
Authors: C. Kirchlechner, N.V. Malyar, N. Peter, C. Liebscher, G. Dehm