Ab initio design of iron-based high-temperature superconductors
Introduction
![Atomic structure of the iron-based super-conductor CaKFe4As4. This compound contains two FeAs-layers separated by a potassium atom in the centre, and calcium atoms at the edges of the unit cell. [2]](/4009839/original-1553262970.jpg?t=eyJ3aWR0aCI6MjQ2LCJvYmpfaWQiOjQwMDk4Mzl9--c57d47996b2fe35f1df6a80199e7589d63cd7f00)
Superconductivity (SC) is one of the most challenging and exotic phenomena in physics since its discovery in the beginning of the last century. Although several approaches have been suggested to explain that effect, only some of the underlying mechanisms are fully understood today. Especially the recently discovered iron-based superconductors (FeSC) are the subject of hot debate.
These materials belong to the group of unconventional high-temperature superconductors, where not a coupling between electrons and lattice vibrations mostly drives the SC, but a direct electron-electron coupling.
The most interesting part for SC is the electronic energy dispersion near the Fermi level. The symmetry of k-space is strongly related to the symmetries of the superconducting wave functions. This region is mainly dominated by the Fe 3d-orbitals and by the 4p-orbitals of the pnictides or chalcogenides [6].
We focus exactly on the interplay of structure and those symmetries. Using ab initio approaches we model different magnetic structures, what is neither possible experimentally nor with effective models, because the interplay of electronic and structural properties is decisive.
Structural Investigations
For mostly all FeSC the structural properties are experimentally well studied, but theoretical not fully explained. Especially for FeSe, FeTe and FeS ab initio approaches are accused to predict less accurate results for the structure in general [6]. Therefore, we put much effort into a more precise and systematic investigation.
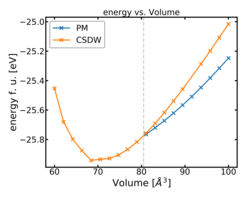
One of the highlights of our approach is the extension of density-functional (DFT) calculations to the paramagnetic state. This is realized by averaging over quasi-random structure to mimic a disordered distribution of spins [5]. Herewith, we transfer methods developed in our group to novel applications. Besides the well-known magnetic influences, we observed that also dipole-dipole interactions can play a significantly role for the internal coupling of the system [7]. Standard DFT calculations only result into a weak coupling between the different Fe-Se layers. Our investigations show that the inclusion of Van-der-Waals (vdW) forces substantially improves the bulk modulus, similar to the behavior observed in graphene. By investigating different implementations of vdW forces into DFT codes, we reveal the mechanisms behind the inter-layer coupling. The combination of both approaches opens a new perspective on magnetic phases and the coupling mechanisms for the superconductivity itself.
Effective Electron Dispersions
The correct description of the structural properties is a prerequisite for an accurate prediction of the electronic dispersion, which is decisive for the determination of the superconducting transition temperature Tc. Coarsening the electronic structure information obtained by DFT, we construct effective tight-binding (TB) models, capturing the symmetric properties of the orbitals of FeSC that have predominantly 3d character. For this purpose, we produce maximally localized Wannier wave functions, which fulfill the orbital character on the one hand and the Bloch symmetry on the other hand.
![Electronic band dispersion of CaKFe4As4 for density-functional calculations [2].](/4010791/original-1553262970.jpg?t=eyJ3aWR0aCI6MjQ2LCJvYmpfaWQiOjQwMTA3OTF9--4b56602bb49a770a5c1f1ebbd909ec4a2d319863)
![Electronic band dispersion of CaKFe4As4 for tight-binding projected bands [2].](/4009931/original-1553262970.jpg?t=eyJ3aWR0aCI6MjQ2LCJvYmpfaWQiOjQwMDk5MzF9--69aa2d918a7dc619abc162d842725c9470a6f02d)
Using this technique, we were able to investigate the symmetry of the superconducting state as well as the influence of the different species for the recently discovered superconductor CaKFe4As4 [2,3].
This material belongs to a completely new structural family called the 1144 compounds. It is especially from a structural point of view interesting. We showed, that the breaking of the gliding symmetry at the Fe-As layer doubles the effective number of hole and electron bands present at the Fermi level. This structural new feature has a strong influence on the superconducting pairing symmetry, such that different non trivial SC wave functions are predicted [2].