Atomic scale investigation of self-healing mechanisms in metallic materials
Motivation
In recent years, much research on the self-healing behavior of materials has been initiated focusing in particular on the recovery of irreversible damage such as cracks or voids. The main idea of the present study is to use the stress-driven martensitic transformation of well-dispersed shape-memory nano-particles inside of a metallic matrix as a self-healing agent. The nano-particles may act as autonomically activated self-healing agents. When a crack tip approaches a nano-particle, the stress field caused by the crack induces a martensitic transformation of the nano-particle. These changes lead to a burst of defects and to strong local stress fields that cause the hindrance of a further crack propagation or the eventual healing of a crack.
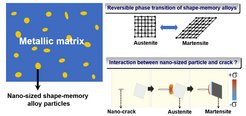
Fig. 1: General concept of designing self-healing metallic materials.
The present study focuses on a specific prototype system (V-Ni-Ti ternary alloy) to demonstrate that dispersed NiTi shape memory nano-particles can indeed improve the ductility of the host matrix phase (V-rich bcc) in a self-healing manner. In particular, atomistic molecular dynamic (MD) simulation that can deal with the martensitic transformation of nano-particles and the fracture behavior of the host matrix phase is an object of concern. Unfortunately, this methodology is limited by the availability of reliable interatomic potentials. Therefore, a hierarchical multi-scale approach, wherein first-principles calculations based upon Density Functional Theory (DFT) are used to inform the atomistic MD simulation, will be performed.
Development of DFT-based interatomic potentials
In the present study, sufficiently accurate interatomic potentials for the V-Ni-Ti ternary systems which will enable simulations of up to several millions of atoms are required. The general strategy of the present research is a bottom-up approach starting from DFT calculations and extending to atomistic simulations employing the interatomic potentials. The most important part in developing interatomic potentials is to reproduce various fundamental physical properties of relevant material’s systems. Extensive DFT calculations of various physical properties are performed to provide necessary information for the optimization and evaluation processes of potential parameters. The target physical properties cover bulk properties (structural energy differences, lattice constants, elastic constants, etc.), defect properties (surface energy, vacancy formation and migration energy, vacancy binding energy, etc.), and thermal properties (melting point, thermal expansion, specific heat, etc.) for pure systems (V, Ti, Ni), binary systems (V-Ti, V-Ni, Ni-Ti) and a ternary system (V-Ni-Ti).
Since a primary stage of the present research is to see the stress-induced martensitic transformation of shape memory nano-particles, a special concern to the properties of transition between austenite (B2 cubic) and martensite (B19’ monoclinic) phases of NiTi shape memory alloy is necessary. However, a tilted angle (~97°) in the martensite phase leads to the crucial dependence on atomic bonding angle and this is very difficult to be realized by simple potential models. Therefore, the second nearest-neighbor modified embedded-atom method (2NN MEAM) [1] that introduces additional angle-dependent terms to consider the directionality of bonds is considered in the present study.
For the fitting process, the general strategy is that the database obtained from DFT calculations and available experimental information will be divided into two sets. One is a training set and the other is a testing set. Such a division allows to estimate the quality and transferability of the target interatomic potential using the testing set which is not included in the fitting process. To optimize the potential parameters, a genetic algorithm code based on the force-matching method is considered.
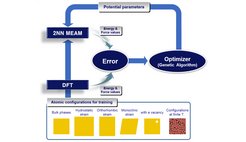
First results
As a first stage, we have developed an interatomic potential for Ni-Ti binary alloy which is the most decisive potential to realize the present project. Fig. 3 shows a temperature-driven reversible phase transition between austenite and martensite phases expected by the present potential. The temperature was initially set to 500 K through thermal equilibration using an isobaric-isothermal (NPT) ensemble MD run with 3-D periodic boundary conditions. Then the thermal load was applied by linearly varying temperature in two stages: (I) cooling from 500 K to 5 K; (II) heating from 5 K to 500 K. The phase transformation was characterized by atomic volume changes. Discontinuous points in the atomic volume versus temperature curve represent phase transformation events. The martensitic start (Ms) and austenite start (As) temperatures are measured to be 185 K and 395 K, respectively.
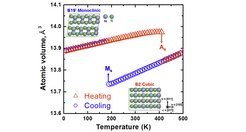
Fig. 3: Atomic volume of the Ni50Ti50 alloy during cooling (blue circles) and heating (red triangles) without external stress. The martensite start (Ms) and austenite start (As) temperatures are indicated by arrows.
Fig. 4(a) presents an atomic configuration of transformed martensite (B19’) phase. As shown, the austenite→martensite transformation undergoes the twinning in the simulation cell with (001) compound twin boundaries which are frequently observed in nanostructured NiTi alloy as shown in Fig 4(b) [2].
Further simulation work was performed to analyze the stress-driven martensitic transformation using the same simulation cell. Among the various possible stress states, the positive hydrostatic stress state was selected to resemble the stress state near a mode-I crack tip under the plain strain condition. The simulations were done for several stress levels, and Fig. 5 shows the resulting phase transition behavior. As can be seen, the martensite start temperature increases under more positive hydrostatic stress. In other words, the martensitic transformation is facilitated by the positive hydrostatic stress at a constant temperature. The result demonstrates that the martensitic transformation of nano-particles by an approaching crack can indeed be realized by atomistic MD simulations using the present potential.
![Fig. 4: (a) Atomic configuration of a twinned martensite (B19’) phase. Twin boundaries are indicated by dashed lines. (b) Atomic structure of nanocrystalline B19’ phase. Experimental HRTEM image of compound twins T1 and T2. (001) twin boundaries and lattice planes of T1 and T2 are indicated by dashed and solid lines, respectively [2].](/3745415/original-1518437933.jpg?t=eyJ3aWR0aCI6MjQ2LCJvYmpfaWQiOjM3NDU0MTV9--cd9fc3506fb26c783cb03cb187be2b7243cf0e79)
Fig. 4: (a) Atomic configuration of a twinned martensite (B19’) phase. Twin boundaries are indicated by dashed lines. (b) Atomic structure of nanocrystalline B19’ phase. Experimental HRTEM image of compound twins T1 and T2. (001) twin boundaries and lattice planes of T1 and T2 are indicated by dashed and solid lines, respectively [2].
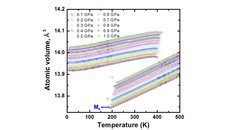
References
Europhys. Lett. 71, 98 (2005)