Grain boundary premelting
Grain boundaries strongly influence the mechanical behavior and other materials properties. At high homologous temperature, grain boundaries can display pronounced disorder, manifested in the most extreme case by the formation of nanometer-scale intergranular films with liquid-like properties. The formation of those films below the bulk melting point, typically referred to as grain boundary premelting, can dramatically reduce shear resistance and lead to catastrophic materials failure.
Grain boundaries strongly influence the mechanical behavior and other materials properties. For this reason, they have been widely studied both experimentally and computationally for decades. At high homologous temperature, grain boundaries can display pronounced disorder, manifested in the most extreme case by the formation of nanometer-scale intergranular films with liquid-like properties. The formation of those films below the bulk melting point, typically referred to as grain boundary premelting, can dramatically reduce shear resistance and lead to catastrophic materials failure. This phenomenon is of interest for predicting the formation of solidification defects associated with the formation of those intergranular films, which can lead to hot cracking during the late stages of solidification, and more generally for understanding the microstructure and mechanical behavior of structural alloys at high homologous temperature.
Grain boundary premelting has been widely studied experimentally as well as theoretically. Theoretical approaches include discrete lattice models and molecular dynamics simulations, as well as conventional phase-field models, which either exploit an orientational order parameter or multiple phase-fields to distinguish between grains, and the phase-field-crystal method, which resolves the crystal density field on an atomic scale and hence naturally models crystal defects such as isolated dislocations and grain boundaries.
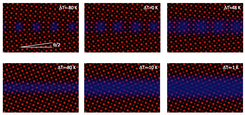
Continuum modeling
We investigate grainboundary premelting and shearing and their relationship using the phase field crystal method and derived amplitude equations. We use the simplest phase field crystal model with the same free-energy function as the Swift-Hohenberg model of pattern formation, which favors hexagonal and bcc ordering in two and three dimensions, respectively. This model can be interpreted as a considerably simplified version of classical density function theory where the crystal density field is dominated by the set of primary reciprocal lattice vectors. It has proven capable of predicting the anisotropic solid-liquid interfacial energy of pure iron and reproducing a subtle dislocation-pairing grain boundary structural transition at high homologous temperatures, previously evidenced in a two-dimensional phase field crystal study of grain boundary premelting, and also observed in Molecular Dynamics simulations.
We have computed quantitatively the disjoining potential for [001] symmetric tilt grain boundaries over the complete range of misorientation and also study quantitatively the response to an applied shear stress, distinguishing between regimes of coupling and sliding. We have also investigated the role of thermal fluctuations on the disjoining potential by carrying out phase field crystal simulations without and with the addition of Langevin noise that is uncorrelated in space and time. To benchmark our results, we have compared the disjoining potentials computed by phase field crystal and Molecular Dynamics simulations. We have also treated the case (analogous to the computation of gamma surfaces) where two crystals of the same orientation are translated from each other in the plane of the interface.